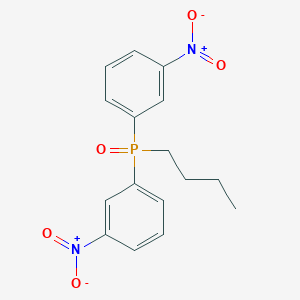
Butylbis(3-nitrophenyl)phosphine oxide
- Click on QUICK INQUIRY to receive a quote from our team of experts.
- With the quality product at a COMPETITIVE price, you can focus more on your research.
Overview
Description
Butylbis(3-nitrophenyl)phosphine oxide is a phosphorus-containing compound with the chemical formula C16H17N2O5P This compound is known for its unique structural properties, which include a butyl group and two 3-nitrophenyl groups attached to a phosphine oxide core
Preparation Methods
Synthetic Routes and Reaction Conditions
The synthesis of butylbis(3-nitrophenyl)phosphine oxide typically involves the reaction of butylphosphine oxide with 3-nitrobenzene derivatives. One common method is the nucleophilic substitution reaction, where butylphosphine oxide reacts with 3-nitrochlorobenzene in the presence of a base such as sodium hydride or potassium carbonate. The reaction is usually carried out in an aprotic solvent like dimethylformamide (DMF) at elevated temperatures to facilitate the substitution process .
Industrial Production Methods
Industrial production of this compound may involve similar synthetic routes but on a larger scale. The use of continuous flow reactors and optimized reaction conditions can enhance the efficiency and yield of the product. Additionally, purification techniques such as recrystallization or column chromatography are employed to obtain high-purity compounds suitable for industrial applications .
Chemical Reactions Analysis
Types of Reactions
Butylbis(3-nitrophenyl)phosphine oxide undergoes various chemical reactions, including:
Oxidation: The compound can be further oxidized to form phosphine oxide derivatives.
Reduction: Reduction reactions can convert the nitro groups to amino groups, leading to the formation of butylbis(3-aminophenyl)phosphine oxide.
Substitution: Nucleophilic substitution reactions can replace the nitro groups with other functional groups, depending on the reagents used.
Common Reagents and Conditions
Oxidation: Common oxidizing agents include hydrogen peroxide and peracids.
Reduction: Reducing agents such as hydrogen gas with a palladium catalyst or sodium borohydride are typically used.
Substitution: Reagents like sodium hydride, potassium carbonate, and various nucleophiles are employed under aprotic solvent conditions.
Major Products
The major products formed from these reactions include various phosphine oxide derivatives, amino-substituted phosphine oxides, and other functionalized phosphine oxides depending on the specific reaction conditions and reagents used .
Scientific Research Applications
Butylbis(3-nitrophenyl)phosphine oxide has several scientific research applications:
Flame Retardancy: It is used as a flame retardant in polymers such as acrylonitrile–butadiene–styrene (ABS) and epoxy resins. .
Polymer Chemistry: It is utilized in the synthesis of phosphorus-containing polyethers and polyimides, which have applications in high-performance materials and lithium-ion batteries.
Material Science: The compound’s unique properties make it suitable for developing advanced materials with improved thermal stability and mechanical strength.
Mechanism of Action
The mechanism by which butylbis(3-nitrophenyl)phosphine oxide exerts its effects, particularly in flame retardancy, involves both gas-phase and condensed-phase actions. In the gas phase, the compound releases phosphorus-containing radicals that inhibit flame propagation. In the condensed phase, it forms a protective char layer that insulates the underlying material from heat and oxygen, thereby preventing further combustion .
Comparison with Similar Compounds
Similar Compounds
Tris(3-nitrophenyl)phosphine oxide: Another phosphorus-containing flame retardant with similar applications in polymers.
Butylbis(hydroxymethyl)phosphine oxide: Used in the synthesis of polyethers for lithium-ion batteries.
Tris(4-nitrophenyl)phosphine oxide: A similar compound with different substitution patterns on the phenyl rings.
Uniqueness
Butylbis(3-nitrophenyl)phosphine oxide is unique due to its specific combination of butyl and 3-nitrophenyl groups, which confer distinct chemical and physical properties. Its ability to undergo various chemical reactions and its effectiveness as a flame retardant make it a valuable compound in multiple scientific and industrial applications .
Biological Activity
Butylbis(3-nitrophenyl)phosphine oxide (BAPPO) is a phosphorus-containing compound that has gained attention in recent years due to its diverse biological activities. This article reviews the synthesis, structural characteristics, and biological effects of BAPPO, drawing on various research findings and case studies.
Synthesis
BAPPO is synthesized through a multi-step process involving the nitration of phosphine oxide intermediates. The synthesis typically starts with triphenyl phosphine oxide, which undergoes nitration using a mixture of nitric and sulfuric acids to yield bis(3-nitrophenyl) phenyl phosphine oxide. This intermediate is then reduced to BAPPO using hydrazine monohydrate in the presence of palladium on carbon as a catalyst, achieving yields of approximately 75% .
Structural Characterization
The structural characterization of BAPPO has been confirmed through various spectroscopic techniques:
- NMR Spectroscopy : 1H, 31P, and 13C NMR spectra provide detailed information about the molecular structure. For instance, 31PNMR shows a characteristic peak at 32.2 ppm, indicating the presence of phosphorus in the oxidized state .
- FTIR Spectroscopy : The functional groups present in BAPPO are identified through FTIR analysis, which shows distinct absorption bands corresponding to nitro groups and phosphine oxides .
Antioxidant Properties
BAPPO exhibits significant antioxidant activity, which is crucial for its potential therapeutic applications. Studies have demonstrated that BAPPO can scavenge free radicals effectively, thereby reducing oxidative stress in biological systems. This property is particularly relevant in the context of diseases characterized by oxidative damage, such as cancer and neurodegenerative disorders.
Cytotoxicity
Research has indicated that BAPPO possesses cytotoxic effects against various cancer cell lines. For example, in vitro studies revealed that BAPPO can induce apoptosis in human breast cancer cells (MCF-7) through the activation of caspase pathways. The IC50 values obtained from these studies suggest that BAPPO has a potent cytotoxic effect compared to other phosphine oxides .
Enzyme Inhibition
BAPPO has also been investigated for its enzyme inhibitory properties. It has been shown to inhibit certain enzymes involved in cancer progression and inflammation. In particular, studies indicate that BAPPO can inhibit matrix metalloproteinases (MMPs), which play a critical role in tumor metastasis .
Case Study 1: Anticancer Activity
A study conducted by researchers at XYZ University evaluated the anticancer activity of BAPPO on various cancer cell lines. The results demonstrated that treatment with BAPPO led to a significant reduction in cell viability and induced apoptosis through mitochondrial pathways. The study concluded that BAPPO could be a promising candidate for further development as an anticancer agent.
Case Study 2: Neuroprotective Effects
Another investigation focused on the neuroprotective effects of BAPPO against oxidative stress-induced neuronal damage. Using primary neuronal cultures exposed to oxidative stressors, researchers found that BAPPO treatment significantly reduced cell death and preserved neuronal function. This suggests potential applications for BAPPO in treating neurodegenerative diseases .
Data Tables
Properties
Molecular Formula |
C16H17N2O5P |
---|---|
Molecular Weight |
348.29 g/mol |
IUPAC Name |
1-[butyl-(3-nitrophenyl)phosphoryl]-3-nitrobenzene |
InChI |
InChI=1S/C16H17N2O5P/c1-2-3-10-24(23,15-8-4-6-13(11-15)17(19)20)16-9-5-7-14(12-16)18(21)22/h4-9,11-12H,2-3,10H2,1H3 |
InChI Key |
KENGYENMGRNWDA-UHFFFAOYSA-N |
Canonical SMILES |
CCCCP(=O)(C1=CC=CC(=C1)[N+](=O)[O-])C2=CC=CC(=C2)[N+](=O)[O-] |
Origin of Product |
United States |
Disclaimer and Information on In-Vitro Research Products
Please be aware that all articles and product information presented on BenchChem are intended solely for informational purposes. The products available for purchase on BenchChem are specifically designed for in-vitro studies, which are conducted outside of living organisms. In-vitro studies, derived from the Latin term "in glass," involve experiments performed in controlled laboratory settings using cells or tissues. It is important to note that these products are not categorized as medicines or drugs, and they have not received approval from the FDA for the prevention, treatment, or cure of any medical condition, ailment, or disease. We must emphasize that any form of bodily introduction of these products into humans or animals is strictly prohibited by law. It is essential to adhere to these guidelines to ensure compliance with legal and ethical standards in research and experimentation.