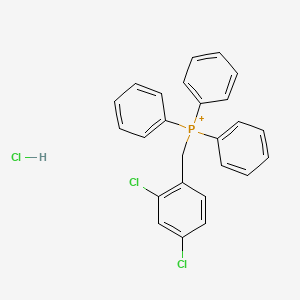
2,4-Dichlorobenzyltriphenylphosphonium chloride
- Click on QUICK INQUIRY to receive a quote from our team of experts.
- With the quality product at a COMPETITIVE price, you can focus more on your research.
Overview
Description
2,4-Dichlorobenzyltriphenylphosphonium chloride is a chemical compound with the molecular formula C25H20Cl3P and a molecular weight of 457.76 g/mol . It is a white to almost white powder or crystalline solid . This compound is used in various scientific research applications due to its unique chemical properties.
Preparation Methods
The synthesis of 2,4-Dichlorobenzyltriphenylphosphonium chloride typically involves the reaction of triphenylphosphine with 2,4-dichlorobenzyl chloride . The reaction is carried out under inert gas conditions to prevent oxidation and moisture interference . The product is then purified through recrystallization to achieve high purity levels . Industrial production methods may involve similar synthetic routes but on a larger scale, with additional steps for purification and quality control .
Chemical Reactions Analysis
2,4-Dichlorobenzyltriphenylphosphonium chloride undergoes various chemical reactions, including:
Substitution Reactions: It can participate in nucleophilic substitution reactions where the chloride ion is replaced by other nucleophiles.
Oxidation and Reduction:
Formation of Wittig Reagents: It is used to form Wittig reagents, which are essential in the synthesis of alkenes from aldehydes and ketones.
Common reagents used in these reactions include strong bases like sodium hydride or potassium tert-butoxide, and solvents such as tetrahydrofuran (THF) or dimethyl sulfoxide (DMSO) . The major products formed depend on the specific reaction conditions and reagents used.
Scientific Research Applications
2,4-Dichlorobenzyltriphenylphosphonium chloride has several scientific research applications:
Mechanism of Action
The mechanism of action of 2,4-Dichlorobenzyltriphenylphosphonium chloride involves its ability to form stable phosphonium ylides, which are key intermediates in the Wittig reaction . These ylides react with carbonyl compounds to form alkenes, a crucial step in many organic synthesis processes . The molecular targets and pathways involved in its biological activities are not well understood and require further research .
Comparison with Similar Compounds
2,4-Dichlorobenzyltriphenylphosphonium chloride can be compared with other similar compounds such as:
2,4-Dichlorobenzyl chloride: A precursor in the synthesis of this compound.
Triphenylphosphine: A reagent used in the synthesis of various phosphonium salts.
2,4-Dichlorobenzenesulfonyl chloride: Another chlorinated benzyl compound with different reactivity and applications.
The uniqueness of this compound lies in its ability to form stable ylides, making it a valuable reagent in organic synthesis .
Biological Activity
2,4-Dichlorobenzyltriphenylphosphonium chloride (DCBTPP) is a phosphonium salt that has garnered attention in various fields, including medicinal chemistry and organic synthesis. Its unique structure, characterized by the presence of a dichlorobenzyl moiety and triphenylphosphonium group, suggests potential biological activities that merit exploration. This article provides a comprehensive overview of the biological activity of DCBTPP, including its mechanisms of action, relevant case studies, and research findings.
Chemical Structure
The chemical structure of DCBTPP can be represented as follows:
This compound features a triphenylphosphonium cation and a 2,4-dichlorobenzyl anion, which contribute to its reactivity and biological properties.
The biological activity of DCBTPP is primarily attributed to its interaction with cellular components. The triphenylphosphonium group is known for its ability to penetrate cellular membranes, potentially influencing mitochondrial function. This characteristic makes it a candidate for studies related to mitochondrial-targeted therapies.
Key Mechanisms:
- Mitochondrial Targeting : DCBTPP may selectively accumulate in mitochondria due to the negative membrane potential.
- Reactive Oxygen Species (ROS) Production : It can induce oxidative stress by increasing ROS levels, which may lead to apoptosis in cancer cells.
- Enzyme Inhibition : DCBTPP has been shown to interact with specific enzymes, modulating their activity and affecting cellular signaling pathways.
Biological Activity
Research has indicated several biological activities associated with DCBTPP:
- Anticancer Activity : Studies have demonstrated that DCBTPP exhibits cytotoxic effects against various cancer cell lines. For instance, it has shown promising results in inhibiting the proliferation of human carcinoma cells.
- Antimicrobial Properties : Preliminary investigations suggest that DCBTPP possesses antimicrobial activity against certain bacterial strains, making it a potential candidate for developing new antimicrobial agents.
- Neuroprotective Effects : There is emerging evidence suggesting that DCBTPP may protect neuronal cells from oxidative damage.
Table 1: Summary of Biological Activities
Case Study: Anticancer Activity
A study conducted by researchers investigated the effects of DCBTPP on human carcinoma cell lines. The results indicated a significant reduction in cell viability at concentrations as low as 10 µM. The mechanism was linked to increased ROS production leading to apoptosis. This study highlights the potential of DCBTPP as an anticancer agent.
Case Study: Antimicrobial Properties
In another investigation focusing on the antimicrobial activity of DCBTPP, researchers tested its efficacy against Gram-positive and Gram-negative bacteria. The compound exhibited notable inhibition zones, particularly against Staphylococcus aureus and Escherichia coli, suggesting its potential as a new antimicrobial agent.
Properties
Molecular Formula |
C25H21Cl3P+ |
---|---|
Molecular Weight |
458.8 g/mol |
IUPAC Name |
(2,4-dichlorophenyl)methyl-triphenylphosphanium;hydrochloride |
InChI |
InChI=1S/C25H20Cl2P.ClH/c26-21-17-16-20(25(27)18-21)19-28(22-10-4-1-5-11-22,23-12-6-2-7-13-23)24-14-8-3-9-15-24;/h1-18H,19H2;1H/q+1; |
InChI Key |
FWBSWSPGFNAXPP-UHFFFAOYSA-N |
Canonical SMILES |
C1=CC=C(C=C1)[P+](CC2=C(C=C(C=C2)Cl)Cl)(C3=CC=CC=C3)C4=CC=CC=C4.Cl |
Origin of Product |
United States |
Disclaimer and Information on In-Vitro Research Products
Please be aware that all articles and product information presented on BenchChem are intended solely for informational purposes. The products available for purchase on BenchChem are specifically designed for in-vitro studies, which are conducted outside of living organisms. In-vitro studies, derived from the Latin term "in glass," involve experiments performed in controlled laboratory settings using cells or tissues. It is important to note that these products are not categorized as medicines or drugs, and they have not received approval from the FDA for the prevention, treatment, or cure of any medical condition, ailment, or disease. We must emphasize that any form of bodily introduction of these products into humans or animals is strictly prohibited by law. It is essential to adhere to these guidelines to ensure compliance with legal and ethical standards in research and experimentation.