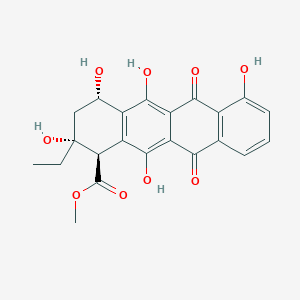
Epsilon-rhodomycinone
Overview
Description
Epsilon-rhodomycinone is a carboxylic ester that is the methyl ester of (1 R ,2 R ,4 S )-2-ethyl-2,4,5,7,12-pentahydroxy-6,11-dioxo-1,2,3,4,6,11-hexahydrotetracene-1-carboxylic acid . It is a metabolite and an antimicrobial agent .
Synthesis Analysis
The biosynthesis of Epsilon-rhodomycinone in Streptomyces peucetius ATCC 27952 is completed in three stages: formation of Epsilon-rhodomycinone, formation of thymidine diphosphate (TDP)-l-daunosamine, and glycosylation followed by post-modifications (methylation, decarboxylation, and hydroxylation) .Molecular Structure Analysis
The molecular structure of Epsilon-rhodomycinone is represented by the chemical formula C22H20O9 .Chemical Reactions Analysis
Epsilon-rhodomycinone can be converted to daunorubicin glycosides by a nonproducing mutant and by a mutant that produces daunorubicin glycosides but not Epsilon-rhodomycinone . The glycosylated product thus formed, then undergoes post-modifications (methylation, decarboxylation, and hydroxylation) to form DXR via DNR .Physical And Chemical Properties Analysis
The physical and chemical properties of Epsilon-rhodomycinone include a molecular weight of 428.38880 and a mono-isotopic mass of 428.11073 .Scientific Research Applications
Anticancer Drug Production
Epsilon-rhodomycinone: is a key intermediate in the biosynthesis of doxorubicin , an anthracycline-type antitumor drug . Doxorubicin is produced by Streptomyces peucetius and is used to treat various cancers, including leukemia, Hodgkin’s lymphoma, and cancers of the bladder, breast, stomach, lung, ovary, thyroid, soft tissue sarcoma, and multiple myeloma . The compound exerts its antiproliferative activity through DNA intercalation and enzyme inhibition, leading to cancer cell death .
Metabolic Engineering
Metabolic engineering strategies have been employed to enhance the production of doxorubicin by manipulating the biosynthetic pathway that includes Epsilon-rhodomycinone . By understanding and applying specific regulatory systems that govern secondary metabolite production, researchers have been able to improve fermentation titers significantly .
Genetic Engineering for Enhanced Production
Genetic engineering techniques have been used to increase the yield of doxorubicin. This involves the modification of the Epsilon-rhodomycinone biosynthesis pathway in Streptomyces peucetius . Through a combination of classical strain mutation and medium optimization, researchers have achieved higher productivity of doxorubicin .
Antibiotic Properties
Beyond its role in anticancer drug synthesis, Rhodomycinone has antibiotic properties. It is a bacterial metabolite found in Streptomyces griseoruber and serves as a precursor to other compounds with antibiotic activity .
Bioconversion Studies
Epsilon-rhodomycinone: is a precursor in the bioconversion process to produce other valuable anthracycline compounds. This includes the conversion to rhodomycin D, which is an intermediate step in the synthesis of daunorubicin and doxorubicin .
Research on Regulatory Systems
The production of Epsilon-rhodomycinone and its subsequent conversion to doxorubicin is tightly regulated. Studies focusing on the regulatory aspects of this biosynthesis can provide insights into the production of other secondary metabolites in Streptomyces species .
Mechanism of Action
Target of Action
Epsilon-rhodomycinone is a type of anthracycline-type polyketide, typically produced by Streptomyces peucetius . It is known to exert antiproliferative activity on cancer cells . .
Mode of Action
The mode of action of Epsilon-rhodomycinone involves two different mechanisms: intercalation and enzyme inhibition . Both of these mechanisms result in DNA disruption that ultimately leads to cell death .
Biochemical Pathways
The biosynthesis of Epsilon-rhodomycinone in Streptomyces peucetius is completed in three stages :
Result of Action
The result of Epsilon-rhodomycinone’s action is the disruption of DNA, which ultimately leads to cell death . This makes it effective against various types of cancer cells .
Action Environment
The action of Epsilon-rhodomycinone can be influenced by various environmental factors. For instance, the production of Epsilon-rhodomycinone by Streptomyces peucetius is tightly regulated, and a very low level of production is maintained in the wild-type strain . .
properties
IUPAC Name |
methyl (1R,2R,4S)-2-ethyl-2,4,5,7,12-pentahydroxy-6,11-dioxo-3,4-dihydro-1H-tetracene-1-carboxylate | |
---|---|---|
Source | PubChem | |
URL | https://pubchem.ncbi.nlm.nih.gov | |
Description | Data deposited in or computed by PubChem | |
InChI |
InChI=1S/C22H20O9/c1-3-22(30)7-10(24)12-13(16(22)21(29)31-2)20(28)14-15(19(12)27)18(26)11-8(17(14)25)5-4-6-9(11)23/h4-6,10,16,23-24,27-28,30H,3,7H2,1-2H3/t10-,16-,22+/m0/s1 | |
Source | PubChem | |
URL | https://pubchem.ncbi.nlm.nih.gov | |
Description | Data deposited in or computed by PubChem | |
InChI Key |
PYFOXRACBORDCT-GOSXWKPOSA-N | |
Source | PubChem | |
URL | https://pubchem.ncbi.nlm.nih.gov | |
Description | Data deposited in or computed by PubChem | |
Canonical SMILES |
CCC1(CC(C2=C(C1C(=O)OC)C(=C3C(=C2O)C(=O)C4=C(C3=O)C=CC=C4O)O)O)O | |
Source | PubChem | |
URL | https://pubchem.ncbi.nlm.nih.gov | |
Description | Data deposited in or computed by PubChem | |
Isomeric SMILES |
CC[C@]1(C[C@@H](C2=C([C@H]1C(=O)OC)C(=C3C(=C2O)C(=O)C4=C(C3=O)C=CC=C4O)O)O)O | |
Source | PubChem | |
URL | https://pubchem.ncbi.nlm.nih.gov | |
Description | Data deposited in or computed by PubChem | |
Molecular Formula |
C22H20O9 | |
Source | PubChem | |
URL | https://pubchem.ncbi.nlm.nih.gov | |
Description | Data deposited in or computed by PubChem | |
Molecular Weight |
428.4 g/mol | |
Source | PubChem | |
URL | https://pubchem.ncbi.nlm.nih.gov | |
Description | Data deposited in or computed by PubChem | |
Product Name |
Epsilon-rhodomycinone | |
CAS RN |
21288-60-8 | |
Record name | Methyl (1R,2R,4S)-2-ethyl-1,2,3,4,6,11-hexahydro-2,4,5,7,12-pentahydroxy-6,11-dioxo-1-naphthacenecarboxylate | |
Source | CAS Common Chemistry | |
URL | https://commonchemistry.cas.org/detail?cas_rn=21288-60-8 | |
Description | CAS Common Chemistry is an open community resource for accessing chemical information. Nearly 500,000 chemical substances from CAS REGISTRY cover areas of community interest, including common and frequently regulated chemicals, and those relevant to high school and undergraduate chemistry classes. This chemical information, curated by our expert scientists, is provided in alignment with our mission as a division of the American Chemical Society. | |
Explanation | The data from CAS Common Chemistry is provided under a CC-BY-NC 4.0 license, unless otherwise stated. | |
Record name | Rhodomycinone | |
Source | ChemIDplus | |
URL | https://pubchem.ncbi.nlm.nih.gov/substance/?source=chemidplus&sourceid=0021288608 | |
Description | ChemIDplus is a free, web search system that provides access to the structure and nomenclature authority files used for the identification of chemical substances cited in National Library of Medicine (NLM) databases, including the TOXNET system. | |
Retrosynthesis Analysis
AI-Powered Synthesis Planning: Our tool employs the Template_relevance Pistachio, Template_relevance Bkms_metabolic, Template_relevance Pistachio_ringbreaker, Template_relevance Reaxys, Template_relevance Reaxys_biocatalysis model, leveraging a vast database of chemical reactions to predict feasible synthetic routes.
One-Step Synthesis Focus: Specifically designed for one-step synthesis, it provides concise and direct routes for your target compounds, streamlining the synthesis process.
Accurate Predictions: Utilizing the extensive PISTACHIO, BKMS_METABOLIC, PISTACHIO_RINGBREAKER, REAXYS, REAXYS_BIOCATALYSIS database, our tool offers high-accuracy predictions, reflecting the latest in chemical research and data.
Strategy Settings
Precursor scoring | Relevance Heuristic |
---|---|
Min. plausibility | 0.01 |
Model | Template_relevance |
Template Set | Pistachio/Bkms_metabolic/Pistachio_ringbreaker/Reaxys/Reaxys_biocatalysis |
Top-N result to add to graph | 6 |
Feasible Synthetic Routes
Q & A
A: Epsilon-rhodomycinone has the molecular formula C21H22O8 and a molecular weight of 402.4 g/mol. []
A: Structural characterization often relies on NMR spectroscopy. For instance, NMR was instrumental in confirming the anomeric purity and configuration of various epsilon-rhodomycinone glycosides. []
A: Epsilon-rhodomycinone is biosynthesized from simpler molecules through a series of enzymatic reactions in certain Streptomyces species. This pathway begins with aklanonic acid, which is methylated, cyclized, and reduced to form aklavinone. Aklavinone is then hydroxylated at the C-11 position by a specific hydroxylase, yielding epsilon-rhodomycinone. []
A: Research suggests that there may be at least two pathways leading to daunorubicin glycosides, with epsilon-rhodomycinone acting as an intermediate in one of these pathways. []
A: Key enzymes include aklanonic acid methyltransferase, aklaviketone reductase, and aklavinone 11-hydroxylase (encoded by the dnrF gene). [, ]
A: Yes, genetic manipulation can significantly impact epsilon-rhodomycinone production. For example, introducing the dnrR1 DNA segment into Streptomyces peucetius resulted in a tenfold increase in epsilon-rhodomycinone production. [] Similarly, disruption of the rhoG gene in Streptomyces violaceus led to the accumulation of epsilon-rhodomycinone and a loss of beta-rhodomycin production. []
A: Yes, epsilon-rhodomycinone can be converted into other anthracyclines through enzymatic modifications. For example, it can be glycosylated to form rhodomycin D by the action of glycosyltransferases. [, ]
A: Glycosylation, the attachment of sugar moieties, is crucial for the biological activity of many anthracyclines, influencing their DNA-binding ability and antitumor activity. []
A: Yes, through a series of enzymatic reactions involving DoxA, DauK, and DauP, epsilon-rhodomycinone glycoside (rhodomycin D) can be converted to doxorubicin. []
A: These anthracyclines intercalate into DNA, disrupting DNA replication and transcription, ultimately leading to cell death. []
A: Research is ongoing to explore the use of epsilon-rhodomycinone and its analogs as potential scaffolds for developing new anticancer drugs with improved efficacy and safety profiles. []
A: Modifications at different positions on the epsilon-rhodomycinone molecule can significantly alter the biological activity of its derivatives. For instance, the introduction of a methyl group at the C-11 position can reduce antitumor activity, as observed with the 11-methyl ether of carminomycin. []
A: The type and configuration of the sugar attached to epsilon-rhodomycinone significantly influence the biological activity of the resulting glycosides. [, ]
A: Epsilon-rhodomycinone is primarily produced by various strains of Streptomyces, including S. peucetius, S. coeruleorubidus, S. violaceus, and S. griseoruber. [, , , , , , , , , , ]
A: Factors such as media composition, carbon source, temperature, pH, and aeration can significantly influence epsilon-rhodomycinone production. Optimization of these parameters is crucial for maximizing yields. [, , , ]
A: Challenges include low production titers, the complexity of fermentation processes, and the potential for strain degeneration. [, ]
Disclaimer and Information on In-Vitro Research Products
Please be aware that all articles and product information presented on BenchChem are intended solely for informational purposes. The products available for purchase on BenchChem are specifically designed for in-vitro studies, which are conducted outside of living organisms. In-vitro studies, derived from the Latin term "in glass," involve experiments performed in controlled laboratory settings using cells or tissues. It is important to note that these products are not categorized as medicines or drugs, and they have not received approval from the FDA for the prevention, treatment, or cure of any medical condition, ailment, or disease. We must emphasize that any form of bodily introduction of these products into humans or animals is strictly prohibited by law. It is essential to adhere to these guidelines to ensure compliance with legal and ethical standards in research and experimentation.