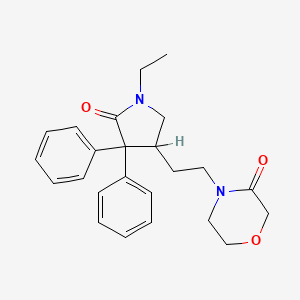
2-Ketodoxapram
Overview
Description
2-Ketodoxapram is a chemical compound that belongs to the class of oxapram derivatives. It is a synthetic compound that has been widely used in scientific research for its potential therapeutic applications. The purpose of
Scientific Research Applications
Pharmacokinetics in Atrial Fibrillation Therapy
2-Ketodoxapram has been studied for its pharmacokinetic properties in the context of atrial fibrillation (AF) therapy. In a study involving porcine models, 2-Ketodoxapram showed a terminal elimination half-life (t1/2) of approximately 2.42 hours and reached a maximal plasma concentration (cmax) of 32.3 ng/mL . This data is crucial for understanding the drug’s behavior in the body and optimizing its therapeutic use for AF.
Respiratory Stimulation in Newborns
Research has compared the effects of doxapram and 2-Ketodoxapram on ventilation in newborn lambs. Both compounds increased minute ventilation, which is a measure of breathing volume. Notably, 2-Ketodoxapram’s effects peaked earlier but also dissipated more quickly than doxapram’s. This suggests potential applications in situations where short-term respiratory stimulation is needed.
Brain Tissue Penetration
The ability of 2-Ketodoxapram to penetrate brain tissue has been evaluated, revealing a brain-to-plasma ratio of 0.12. This indicates that the compound can cross the blood-brain barrier, albeit to a limited extent, which could be relevant for central nervous system disorders .
Protein Binding Affinity
2-Ketodoxapram exhibits a high degree of protein binding (98.4%), which affects its distribution, efficacy, and clearance from the body . Understanding this property is essential for drug formulation and predicting interactions with other medications.
Development of Analytical Assays
The development of ultra-performance liquid chromatography tandem mass spectrometry (UPLC-MS/MS) assays for 2-Ketodoxapram has been a significant application. Such assays are vital for quantifying the compound in biological samples, which is a fundamental step in pharmacokinetic studies .
Safety Profile Enhancement
The pharmacokinetic data obtained from studies using 2-Ketodoxapram can contribute to enhancing the safety profile of doxapram’s usage. By understanding the metabolite’s behavior in the body, researchers can better predict and mitigate potential adverse effects .
properties
IUPAC Name |
4-[2-(1-ethyl-5-oxo-4,4-diphenylpyrrolidin-3-yl)ethyl]morpholin-3-one | |
---|---|---|
Source | PubChem | |
URL | https://pubchem.ncbi.nlm.nih.gov | |
Description | Data deposited in or computed by PubChem | |
InChI |
InChI=1S/C24H28N2O3/c1-2-25-17-21(13-14-26-15-16-29-18-22(26)27)24(23(25)28,19-9-5-3-6-10-19)20-11-7-4-8-12-20/h3-12,21H,2,13-18H2,1H3 | |
Source | PubChem | |
URL | https://pubchem.ncbi.nlm.nih.gov | |
Description | Data deposited in or computed by PubChem | |
InChI Key |
LLCHHQQQMSDZLP-UHFFFAOYSA-N | |
Source | PubChem | |
URL | https://pubchem.ncbi.nlm.nih.gov | |
Description | Data deposited in or computed by PubChem | |
Canonical SMILES |
CCN1CC(C(C1=O)(C2=CC=CC=C2)C3=CC=CC=C3)CCN4CCOCC4=O | |
Source | PubChem | |
URL | https://pubchem.ncbi.nlm.nih.gov | |
Description | Data deposited in or computed by PubChem | |
Molecular Formula |
C24H28N2O3 | |
Source | PubChem | |
URL | https://pubchem.ncbi.nlm.nih.gov | |
Description | Data deposited in or computed by PubChem | |
DSSTOX Substance ID |
DTXSID80962591 | |
Record name | 4-[2-(1-Ethyl-5-oxo-4,4-diphenylpyrrolidin-3-yl)ethyl]morpholin-3-one | |
Source | EPA DSSTox | |
URL | https://comptox.epa.gov/dashboard/DTXSID80962591 | |
Description | DSSTox provides a high quality public chemistry resource for supporting improved predictive toxicology. | |
Molecular Weight |
392.5 g/mol | |
Source | PubChem | |
URL | https://pubchem.ncbi.nlm.nih.gov | |
Description | Data deposited in or computed by PubChem | |
CAS RN |
42595-88-0 | |
Record name | 2-Ketodoxapram | |
Source | ChemIDplus | |
URL | https://pubchem.ncbi.nlm.nih.gov/substance/?source=chemidplus&sourceid=0042595880 | |
Description | ChemIDplus is a free, web search system that provides access to the structure and nomenclature authority files used for the identification of chemical substances cited in National Library of Medicine (NLM) databases, including the TOXNET system. | |
Record name | 4-[2-(1-Ethyl-5-oxo-4,4-diphenylpyrrolidin-3-yl)ethyl]morpholin-3-one | |
Source | EPA DSSTox | |
URL | https://comptox.epa.gov/dashboard/DTXSID80962591 | |
Description | DSSTox provides a high quality public chemistry resource for supporting improved predictive toxicology. | |
Record name | 4-[2-(1-ethyl-5-oxo-4,4-diphenylpyrrolidin-3-yl)ethyl]morpholin-3-one | |
Source | European Chemicals Agency (ECHA) | |
URL | https://echa.europa.eu/information-on-chemicals | |
Description | The European Chemicals Agency (ECHA) is an agency of the European Union which is the driving force among regulatory authorities in implementing the EU's groundbreaking chemicals legislation for the benefit of human health and the environment as well as for innovation and competitiveness. | |
Explanation | Use of the information, documents and data from the ECHA website is subject to the terms and conditions of this Legal Notice, and subject to other binding limitations provided for under applicable law, the information, documents and data made available on the ECHA website may be reproduced, distributed and/or used, totally or in part, for non-commercial purposes provided that ECHA is acknowledged as the source: "Source: European Chemicals Agency, http://echa.europa.eu/". Such acknowledgement must be included in each copy of the material. ECHA permits and encourages organisations and individuals to create links to the ECHA website under the following cumulative conditions: Links can only be made to webpages that provide a link to the Legal Notice page. | |
Record name | AHR-5955 | |
Source | FDA Global Substance Registration System (GSRS) | |
URL | https://gsrs.ncats.nih.gov/ginas/app/beta/substances/7SY5VUG7T7 | |
Description | The FDA Global Substance Registration System (GSRS) enables the efficient and accurate exchange of information on what substances are in regulated products. Instead of relying on names, which vary across regulatory domains, countries, and regions, the GSRS knowledge base makes it possible for substances to be defined by standardized, scientific descriptions. | |
Explanation | Unless otherwise noted, the contents of the FDA website (www.fda.gov), both text and graphics, are not copyrighted. They are in the public domain and may be republished, reprinted and otherwise used freely by anyone without the need to obtain permission from FDA. Credit to the U.S. Food and Drug Administration as the source is appreciated but not required. | |
Retrosynthesis Analysis
AI-Powered Synthesis Planning: Our tool employs the Template_relevance Pistachio, Template_relevance Bkms_metabolic, Template_relevance Pistachio_ringbreaker, Template_relevance Reaxys, Template_relevance Reaxys_biocatalysis model, leveraging a vast database of chemical reactions to predict feasible synthetic routes.
One-Step Synthesis Focus: Specifically designed for one-step synthesis, it provides concise and direct routes for your target compounds, streamlining the synthesis process.
Accurate Predictions: Utilizing the extensive PISTACHIO, BKMS_METABOLIC, PISTACHIO_RINGBREAKER, REAXYS, REAXYS_BIOCATALYSIS database, our tool offers high-accuracy predictions, reflecting the latest in chemical research and data.
Strategy Settings
Precursor scoring | Relevance Heuristic |
---|---|
Min. plausibility | 0.01 |
Model | Template_relevance |
Template Set | Pistachio/Bkms_metabolic/Pistachio_ringbreaker/Reaxys/Reaxys_biocatalysis |
Top-N result to add to graph | 6 |
Feasible Synthetic Routes
Q & A
Q1: What is the significance of studying 2-ketodoxapram alongside doxapram?
A1: Doxapram is metabolized in the body to form 2-ketodoxapram. [] Understanding the pharmacokinetics of both the parent drug and its active metabolite is crucial because 2-ketodoxapram may contribute to the overall therapeutic effect of doxapram. Studying both compounds allows researchers to assess their individual contributions to efficacy and potential side effects.
Q2: What analytical methods have been developed to study the pharmacokinetics of doxapram and 2-ketodoxapram?
A2: Researchers have developed a highly sensitive and specific ultra-performance liquid chromatography tandem mass spectrometry (UPLC-MS/MS) assay to simultaneously quantify doxapram and 2-ketodoxapram in biological samples. [] This method allows for accurate measurement of both compounds in plasma and tissues, enabling comprehensive pharmacokinetic studies. This method demonstrated a low limit of quantification for both doxapram and 2-ketodoxapram in both plasma and brain tissue. [] Additionally, a gas chromatography method has been developed for the simultaneous determination of doxapram and 2-ketodoxapram specifically in the plasma of neonates. []
Disclaimer and Information on In-Vitro Research Products
Please be aware that all articles and product information presented on BenchChem are intended solely for informational purposes. The products available for purchase on BenchChem are specifically designed for in-vitro studies, which are conducted outside of living organisms. In-vitro studies, derived from the Latin term "in glass," involve experiments performed in controlled laboratory settings using cells or tissues. It is important to note that these products are not categorized as medicines or drugs, and they have not received approval from the FDA for the prevention, treatment, or cure of any medical condition, ailment, or disease. We must emphasize that any form of bodily introduction of these products into humans or animals is strictly prohibited by law. It is essential to adhere to these guidelines to ensure compliance with legal and ethical standards in research and experimentation.