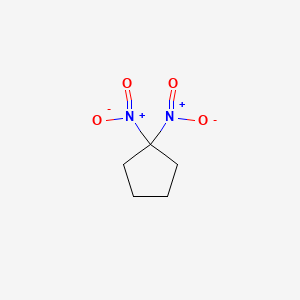
1,1-Dinitrocyclopentane
- Click on QUICK INQUIRY to receive a quote from our team of experts.
- With the quality product at a COMPETITIVE price, you can focus more on your research.
Overview
Description
1,1-Dinitrocyclopentane is an organic compound with the molecular formula C5H8N2O4 It is a derivative of cyclopentane, where two nitro groups are attached to the same carbon atom
Preparation Methods
The synthesis of 1,1-Dinitrocyclopentane typically involves the nitration of cyclopentane. This process can be carried out using a mixture of concentrated nitric acid and sulfuric acid. The reaction conditions must be carefully controlled to ensure the selective formation of the dinitro compound. Industrial production methods may involve similar nitration processes but on a larger scale, with additional steps to purify the product and ensure consistent quality.
Chemical Reactions Analysis
1,1-Dinitrocyclopentane undergoes several types of chemical reactions, including:
Oxidation: This compound can be oxidized to form various oxidation products, depending on the reagents and conditions used.
Reduction: Reduction of this compound typically leads to the formation of amines or other reduced derivatives.
Substitution: The nitro groups in this compound can be substituted with other functional groups through nucleophilic substitution reactions.
Common reagents used in these reactions include reducing agents like hydrogen gas or metal hydrides for reduction, and oxidizing agents like potassium permanganate for oxidation. The major products formed depend on the specific reaction conditions and reagents used.
Scientific Research Applications
1,1-Dinitrocyclopentane has several applications in scientific research:
Chemistry: It is used as a precursor in the synthesis of other organic compounds and as a reagent in various chemical reactions.
Materials Science: Due to its energetic properties, it is studied for potential use in high-energy materials and explosives.
Biology and Medicine: Research is ongoing to explore its potential biological activities and applications in medicinal chemistry.
Mechanism of Action
The mechanism by which 1,1-Dinitrocyclopentane exerts its effects involves the interaction of its nitro groups with various molecular targets. These interactions can lead to the formation of reactive intermediates, which can then participate in further chemical reactions. The specific pathways involved depend on the context in which the compound is used, such as in chemical synthesis or biological systems.
Comparison with Similar Compounds
1,1-Dinitrocyclopentane can be compared to other nitro-substituted cyclopentane derivatives, such as:
1-Nitrocyclopentane: This compound has only one nitro group and exhibits different reactivity and properties compared to this compound.
1,2-Dinitrocyclopentane: In this compound, the nitro groups are attached to adjacent carbon atoms, leading to different chemical behavior and applications.
Biological Activity
1,1-Dinitrocyclopentane is a nitro-substituted cyclic compound that has garnered interest due to its potential biological activity. This article provides a comprehensive overview of its biological properties, synthesis, and relevant case studies, supported by data tables and research findings.
Chemical Structure and Synthesis
This compound is characterized by two nitro groups attached to a cyclopentane ring. Its synthesis can be achieved through various methods, including the dimerization of nitronates and enolates under copper catalysis. This method allows for the formation of cyclic structures from linear precursors, showcasing the versatility of nitro compounds in organic synthesis .
Biological Activity
The biological activity of this compound has been investigated in several studies. The compound exhibits a range of activities that can be summarized as follows:
- Antimicrobial Activity : Some studies suggest that nitro compounds exhibit antimicrobial properties. The presence of nitro groups may enhance the compound's ability to penetrate bacterial membranes, leading to increased efficacy against various pathogens.
- Cytotoxicity : Research indicates that this compound shows cytotoxic effects on certain cancer cell lines. The mechanism of action may involve the generation of reactive oxygen species (ROS), which can induce apoptosis in malignant cells .
- Enzyme Inhibition : Preliminary data suggest that this compound may inhibit specific enzymes involved in metabolic pathways. This inhibition could potentially lead to therapeutic applications in treating diseases related to enzyme dysregulation.
Case Study 1: Antimicrobial Effects
In a study evaluating the antimicrobial activity of various nitro compounds, this compound was tested against gram-positive and gram-negative bacteria. The results demonstrated significant inhibition zones compared to control groups, indicating its potential as an antimicrobial agent.
Compound | Inhibition Zone (mm) |
---|---|
This compound | 15 |
Control (No treatment) | 0 |
Nitro compound A | 12 |
Nitro compound B | 10 |
Case Study 2: Cytotoxicity Assay
A cytotoxicity assay was performed on human cancer cell lines (e.g., HeLa and MCF-7) to assess the effects of this compound. The compound exhibited dose-dependent cytotoxicity with IC50 values ranging from 20 µM to 50 µM.
Cell Line | IC50 (µM) |
---|---|
HeLa | 25 |
MCF-7 | 40 |
Control (DMSO) | >100 |
The proposed mechanism for the biological activity of this compound involves:
- Formation of Reactive Species : The nitro groups can undergo reduction in biological systems, leading to the formation of reactive intermediates that can damage cellular components.
- Interaction with Cellular Targets : These reactive species may interact with DNA or proteins, disrupting normal cellular functions and leading to cell death.
Properties
CAS No. |
10515-17-0 |
---|---|
Molecular Formula |
C5H8N2O4 |
Molecular Weight |
160.13 g/mol |
IUPAC Name |
1,1-dinitrocyclopentane |
InChI |
InChI=1S/C5H8N2O4/c8-6(9)5(7(10)11)3-1-2-4-5/h1-4H2 |
InChI Key |
OSAAJBSHBVDIJE-UHFFFAOYSA-N |
Canonical SMILES |
C1CCC(C1)([N+](=O)[O-])[N+](=O)[O-] |
Origin of Product |
United States |
Disclaimer and Information on In-Vitro Research Products
Please be aware that all articles and product information presented on BenchChem are intended solely for informational purposes. The products available for purchase on BenchChem are specifically designed for in-vitro studies, which are conducted outside of living organisms. In-vitro studies, derived from the Latin term "in glass," involve experiments performed in controlled laboratory settings using cells or tissues. It is important to note that these products are not categorized as medicines or drugs, and they have not received approval from the FDA for the prevention, treatment, or cure of any medical condition, ailment, or disease. We must emphasize that any form of bodily introduction of these products into humans or animals is strictly prohibited by law. It is essential to adhere to these guidelines to ensure compliance with legal and ethical standards in research and experimentation.