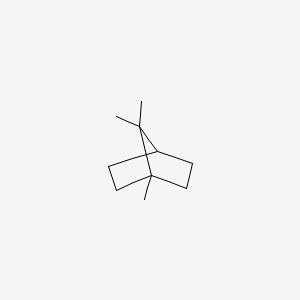
Camphane
Overview
Description
Camphane, also known as 2,2-dimethyl-3-methylenebicyclo[2.2.1]heptane, is a bicyclic monoterpene. It is a naturally occurring compound found in various essential oils, including turpentine, cypress oil, camphor oil, citronella oil, and neroli . This compound is colorless, crystalline, and has a camphor-like smell. It is insoluble in water but soluble in organic solvents such as ethanol, diethyl ether, and chloroform .
Preparation Methods
Synthetic Routes and Reaction Conditions
Camphane can be synthesized from α-pinene through isomerization using a solid acid catalyst such as titanium dioxide . The process involves the conversion of α-pinene to isobornyl ester, which is then hydrolyzed and oxidized to produce this compound .
Industrial Production Methods
Industrial production of this compound typically involves the isomerization of α-pinene, a common monoterpene found in turpentine oil. The process uses solid acid catalysts to achieve high yields of this compound .
Chemical Reactions Analysis
Types of Reactions
Camphane undergoes various chemical reactions, including:
Oxidation: This compound can be oxidized to form camphor and other oxygenated derivatives.
Reduction: Reduction of this compound can yield isoborneol and borneol.
Common Reagents and Conditions
Oxidation: Common oxidizing agents include potassium permanganate and chromium trioxide.
Reduction: Reducing agents such as lithium aluminum hydride and sodium borohydride are used.
Substitution: Catalysts like BF3·Et2O or ZnCl2 are employed for electrophilic addition reactions.
Major Products Formed
Oxidation: Camphor and other oxygenated derivatives.
Reduction: Isoborneol and borneol.
Substitution: Various thiol-substituted this compound derivatives.
Scientific Research Applications
Camphane has diverse applications in scientific research, including:
Chemistry: Used as a starting material for the synthesis of various organic compounds.
Biology: Investigated for its potential antioxidant, anti-inflammatory, and antimicrobial properties.
Medicine: Explored for its hypolipidemic effects, particularly in reducing cholesterol and triglycerides.
Industry: Utilized in the fragrance and flavor industries due to its distinctive aroma and taste.
Mechanism of Action
Camphane exerts its hypolipidemic action by affecting the expression of sterol regulatory element-binding protein 1 (SREBP-1) and microsomal triglyceride transfer protein (MTP) . It inhibits the biosynthesis of cholesterol and triglycerides in a concentration-dependent manner, leading to reduced levels of these lipids in the plasma . The mechanism involves the upregulation of SREBP-1 expression and inhibition of MTP, which are crucial for lipid metabolism .
Comparison with Similar Compounds
Similar Compounds
Camphor: An oxygenated derivative of camphane with a similar structure but different functional groups.
Isoborneol: A reduction product of this compound with a hydroxyl group.
Borneol: Another reduction product of this compound, isomeric with isoborneol.
Uniqueness of this compound
This compound is unique due to its bicyclic structure and its ability to undergo various chemical transformations, making it a versatile compound in organic synthesis . Its distinct aroma and potential therapeutic properties further distinguish it from other similar compounds .
Properties
IUPAC Name |
1,7,7-trimethylbicyclo[2.2.1]heptane | |
---|---|---|
Source | PubChem | |
URL | https://pubchem.ncbi.nlm.nih.gov | |
Description | Data deposited in or computed by PubChem | |
InChI |
InChI=1S/C10H18/c1-9(2)8-4-6-10(9,3)7-5-8/h8H,4-7H2,1-3H3 | |
Source | PubChem | |
URL | https://pubchem.ncbi.nlm.nih.gov | |
Description | Data deposited in or computed by PubChem | |
InChI Key |
BEWYHVAWEKZDPP-UHFFFAOYSA-N | |
Source | PubChem | |
URL | https://pubchem.ncbi.nlm.nih.gov | |
Description | Data deposited in or computed by PubChem | |
Canonical SMILES |
CC1(C2CCC1(CC2)C)C | |
Source | PubChem | |
URL | https://pubchem.ncbi.nlm.nih.gov | |
Description | Data deposited in or computed by PubChem | |
Molecular Formula |
C10H18 | |
Source | PubChem | |
URL | https://pubchem.ncbi.nlm.nih.gov | |
Description | Data deposited in or computed by PubChem | |
DSSTOX Substance ID |
DTXSID60196832 | |
Record name | Camphane | |
Source | EPA DSSTox | |
URL | https://comptox.epa.gov/dashboard/DTXSID60196832 | |
Description | DSSTox provides a high quality public chemistry resource for supporting improved predictive toxicology. | |
Molecular Weight |
138.25 g/mol | |
Source | PubChem | |
URL | https://pubchem.ncbi.nlm.nih.gov | |
Description | Data deposited in or computed by PubChem | |
CAS No. |
464-15-3 | |
Record name | Bornane | |
Source | CAS Common Chemistry | |
URL | https://commonchemistry.cas.org/detail?cas_rn=464-15-3 | |
Description | CAS Common Chemistry is an open community resource for accessing chemical information. Nearly 500,000 chemical substances from CAS REGISTRY cover areas of community interest, including common and frequently regulated chemicals, and those relevant to high school and undergraduate chemistry classes. This chemical information, curated by our expert scientists, is provided in alignment with our mission as a division of the American Chemical Society. | |
Explanation | The data from CAS Common Chemistry is provided under a CC-BY-NC 4.0 license, unless otherwise stated. | |
Record name | Camphane | |
Source | ChemIDplus | |
URL | https://pubchem.ncbi.nlm.nih.gov/substance/?source=chemidplus&sourceid=0000464153 | |
Description | ChemIDplus is a free, web search system that provides access to the structure and nomenclature authority files used for the identification of chemical substances cited in National Library of Medicine (NLM) databases, including the TOXNET system. | |
Record name | Camphane | |
Source | DrugBank | |
URL | https://www.drugbank.ca/drugs/DB04501 | |
Description | The DrugBank database is a unique bioinformatics and cheminformatics resource that combines detailed drug (i.e. chemical, pharmacological and pharmaceutical) data with comprehensive drug target (i.e. sequence, structure, and pathway) information. | |
Explanation | Creative Common's Attribution-NonCommercial 4.0 International License (http://creativecommons.org/licenses/by-nc/4.0/legalcode) | |
Record name | Camphane | |
Source | DTP/NCI | |
URL | https://dtp.cancer.gov/dtpstandard/servlet/dwindex?searchtype=NSC&outputformat=html&searchlist=17531 | |
Description | The NCI Development Therapeutics Program (DTP) provides services and resources to the academic and private-sector research communities worldwide to facilitate the discovery and development of new cancer therapeutic agents. | |
Explanation | Unless otherwise indicated, all text within NCI products is free of copyright and may be reused without our permission. Credit the National Cancer Institute as the source. | |
Record name | Camphane | |
Source | EPA DSSTox | |
URL | https://comptox.epa.gov/dashboard/DTXSID60196832 | |
Description | DSSTox provides a high quality public chemistry resource for supporting improved predictive toxicology. | |
Retrosynthesis Analysis
AI-Powered Synthesis Planning: Our tool employs the Template_relevance Pistachio, Template_relevance Bkms_metabolic, Template_relevance Pistachio_ringbreaker, Template_relevance Reaxys, Template_relevance Reaxys_biocatalysis model, leveraging a vast database of chemical reactions to predict feasible synthetic routes.
One-Step Synthesis Focus: Specifically designed for one-step synthesis, it provides concise and direct routes for your target compounds, streamlining the synthesis process.
Accurate Predictions: Utilizing the extensive PISTACHIO, BKMS_METABOLIC, PISTACHIO_RINGBREAKER, REAXYS, REAXYS_BIOCATALYSIS database, our tool offers high-accuracy predictions, reflecting the latest in chemical research and data.
Strategy Settings
Precursor scoring | Relevance Heuristic |
---|---|
Min. plausibility | 0.01 |
Model | Template_relevance |
Template Set | Pistachio/Bkms_metabolic/Pistachio_ringbreaker/Reaxys/Reaxys_biocatalysis |
Top-N result to add to graph | 6 |
Feasible Synthetic Routes
Disclaimer and Information on In-Vitro Research Products
Please be aware that all articles and product information presented on BenchChem are intended solely for informational purposes. The products available for purchase on BenchChem are specifically designed for in-vitro studies, which are conducted outside of living organisms. In-vitro studies, derived from the Latin term "in glass," involve experiments performed in controlled laboratory settings using cells or tissues. It is important to note that these products are not categorized as medicines or drugs, and they have not received approval from the FDA for the prevention, treatment, or cure of any medical condition, ailment, or disease. We must emphasize that any form of bodily introduction of these products into humans or animals is strictly prohibited by law. It is essential to adhere to these guidelines to ensure compliance with legal and ethical standards in research and experimentation.