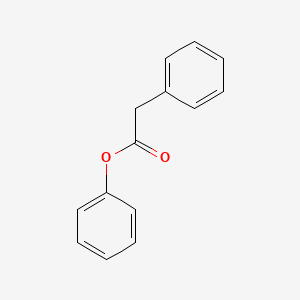
Phenyl phenylacetate
Overview
Description
Phenyl phenylacetate is an organic compound that belongs to the ester class of chemicals. It is formed by the esterification of phenylacetic acid and phenol. This compound is known for its pleasant aroma and is often used in the fragrance and flavor industry. Its chemical structure consists of a phenyl group attached to the carbonyl carbon of the ester, which is further connected to another phenyl group.
Mechanism of Action
Target of Action
Phenyl phenylacetate, also known as phenylacetic acid (PAA), is a central intermediate metabolite involved in bacterial degradation of aromatic components . The primary targets of this compound are the enzymes involved in the bacterial PAA pathway . These enzymes play a crucial role in biofilm formation and antimicrobial activity .
Mode of Action
This compound interacts with its targets, the enzymes in the bacterial PAA pathway, to mediate the degradation of aromatic components . This interaction results in changes in the bacterial metabolism, affecting biofilm formation and antimicrobial activity .
Biochemical Pathways
The biochemical pathway affected by this compound is the bacterial PAA pathway . This pathway mainly contains 12 enzymes and a transcriptional regulator . The strategy of this pathway is exceptional in several aspects. Intermediates are processed as CoA thioesters, and the aromatic ring of phenylacetyl-CoA becomes activated to a ring 1,2-epoxide by a distinct multicomponent oxygenase . This isomerization is followed by hydrolytic ring cleavage and β-oxidation steps, leading to acetyl-CoA and succinyl-CoA .
Pharmacokinetics
This compound exhibits nonlinear pharmacokinetics . The clearance of this compound was found to be 60-66 ml/min per m² . The half-life of this compound was found to be 55-77 minutes . The volume of distribution for this compound was found to be 7.9±3.4 l/m² .
Result of Action
The molecular and cellular effects of this compound’s action include growth inhibition and modulation of differentiation in various tumor cell lines . The growth inhibition was cytostatic with the cells arrested in the G0–1 cell phase .
Action Environment
The action of this compound is influenced by environmental factors such as the availability of oxygen . Aerobic pathways use oxygen both for hydroxylation and for cleavage of the ring . In contrast, under anaerobic conditions, the common strategy consists of activation by CoA-thioester formation, shortening of the side chain, and energy-driven ring reduction .
Biochemical Analysis
Biochemical Properties
Phenyl phenylacetate plays a crucial role in biochemical reactions, particularly in the catabolism of aromatic compounds. It is involved in the phenylacetate catabolic pathway, where it is initially transformed into phenylacetyl-CoA. This transformation is facilitated by the enzyme phenylacetate-CoA ligase. This compound interacts with various enzymes, including multicomponent oxygenases, which hydroxylate phenylacetyl-CoA to form dihydrodiol derivatives. These interactions are essential for the subsequent steps in the catabolic pathway, leading to the breakdown of the aromatic ring and the formation of acetyl-CoA and succinyl-CoA .
Cellular Effects
This compound influences various cellular processes, including cell signaling pathways, gene expression, and cellular metabolism. In bacterial cells, this compound is involved in the degradation of aromatic compounds, which is crucial for the utilization of these compounds as carbon sources. This compound affects the expression of genes involved in the phenylacetate catabolic pathway, thereby regulating the metabolic flux through this pathway. Additionally, this compound can impact cellular metabolism by altering the levels of key metabolites such as acetyl-CoA and succinyl-CoA .
Molecular Mechanism
The molecular mechanism of this compound involves its conversion to phenylacetyl-CoA by phenylacetate-CoA ligase. This conversion is followed by a series of enzymatic reactions, including hydroxylation by multicomponent oxygenases, which produce dihydrodiol derivatives. These derivatives undergo further transformations, leading to the cleavage of the aromatic ring and the formation of acetyl-CoA and succinyl-CoA. The binding interactions of this compound with enzymes such as phenylacetate-CoA ligase and multicomponent oxygenases are critical for its biochemical activity .
Temporal Effects in Laboratory Settings
In laboratory settings, the effects of this compound can change over time due to its stability and degradation. Studies have shown that this compound is relatively stable under aerobic conditions, allowing for sustained biochemical activity. Under anaerobic conditions, the degradation of this compound can lead to the accumulation of intermediate compounds, which may have long-term effects on cellular function. These temporal effects are important for understanding the dynamics of this compound in various experimental settings .
Dosage Effects in Animal Models
The effects of this compound vary with different dosages in animal models. At low doses, this compound can enhance the catabolism of aromatic compounds, promoting metabolic activity. At high doses, this compound may exhibit toxic effects, leading to cellular damage and adverse physiological responses. Studies have identified threshold effects, where the beneficial effects of this compound are observed at specific dosage ranges, beyond which toxicity becomes apparent .
Metabolic Pathways
This compound is involved in the phenylacetate catabolic pathway, which includes several key enzymes and cofactors. The initial step involves the conversion of this compound to phenylacetyl-CoA by phenylacetate-CoA ligase. This is followed by hydroxylation reactions catalyzed by multicomponent oxygenases, leading to the formation of dihydrodiol derivatives. These intermediates undergo further enzymatic transformations, resulting in the production of acetyl-CoA and succinyl-CoA. The metabolic flux through this pathway is regulated by the availability of this compound and the activity of the involved enzymes .
Transport and Distribution
This compound is transported and distributed within cells and tissues through specific transporters and binding proteins. These transporters facilitate the uptake of this compound into cells, where it can interact with enzymes involved in its catabolic pathway. The distribution of this compound within cellular compartments is crucial for its biochemical activity, as it needs to be localized to specific sites for effective interaction with enzymes such as phenylacetate-CoA ligase .
Subcellular Localization
The subcellular localization of this compound is determined by targeting signals and post-translational modifications that direct it to specific compartments or organelles. This compound is primarily localized in the cytoplasm, where it interacts with enzymes involved in its catabolic pathway. This localization is essential for its activity, as it ensures that this compound is available for conversion to phenylacetyl-CoA and subsequent enzymatic reactions .
Preparation Methods
Synthetic Routes and Reaction Conditions: Phenyl phenylacetate can be synthesized through several methods:
Esterification of Phenylacetic Acid and Phenol: This is the most common method. The reaction involves heating phenylacetic acid with phenol in the presence of a catalyst such as sulfuric acid or hydrochloric acid. The reaction is typically carried out under reflux conditions to ensure complete esterification.
Use of Acetic Anhydride: Another method involves the reaction of phenol with acetic anhydride in the presence of a base like pyridine. This method is advantageous as it avoids the use of strong acids and can be carried out at lower temperatures.
Industrial Production Methods: In industrial settings, this compound is often produced using continuous flow reactors to ensure consistent quality and yield. The use of ionic liquids as catalysts has also been explored to make the process more environmentally friendly and cost-effective .
Chemical Reactions Analysis
Phenyl phenylacetate undergoes various chemical reactions, including:
Hydrolysis: In the presence of water and an acid or base catalyst, this compound can be hydrolyzed back to phenylacetic acid and phenol.
Reduction: It can be reduced to this compound alcohol using reducing agents like lithium aluminum hydride.
Substitution Reactions: The ester group can be substituted by other nucleophiles, leading to the formation of different derivatives.
Common Reagents and Conditions:
Hydrolysis: Acidic or basic conditions with water.
Reduction: Lithium aluminum hydride or sodium borohydride.
Substitution: Nucleophiles like amines or alcohols under mild conditions.
Major Products Formed:
Hydrolysis: Phenylacetic acid and phenol.
Reduction: this compound alcohol.
Substitution: Various ester derivatives depending on the nucleophile used.
Scientific Research Applications
Phenyl phenylacetate has several applications in scientific research:
Chemistry: It is used as an intermediate in the synthesis of various organic compounds.
Biology: It serves as a model compound in studies involving ester hydrolysis and enzyme catalysis.
Medicine: Research is being conducted on its potential use in drug delivery systems due to its ability to form stable esters.
Industry: It is widely used in the fragrance and flavor industry due to its pleasant aroma.
Comparison with Similar Compounds
Phenyl phenylacetate can be compared with other similar esters such as:
Methyl Phenylacetate: Similar in structure but has a methyl group instead of a phenyl group. It is also used in fragrances but has a different aroma profile.
Ethyl Phenylacetate: Contains an ethyl group and is used in flavorings and fragrances.
Phenethyl Phenylacetate: Has an additional ethyl group on the phenyl ring, leading to different chemical properties and applications.
Uniqueness: this compound is unique due to its dual phenyl groups, which contribute to its distinct aroma and chemical reactivity. This makes it particularly valuable in the fragrance industry and as a model compound in scientific research.
Properties
IUPAC Name |
phenyl 2-phenylacetate | |
---|---|---|
Source | PubChem | |
URL | https://pubchem.ncbi.nlm.nih.gov | |
Description | Data deposited in or computed by PubChem | |
InChI |
InChI=1S/C14H12O2/c15-14(11-12-7-3-1-4-8-12)16-13-9-5-2-6-10-13/h1-10H,11H2 | |
Source | PubChem | |
URL | https://pubchem.ncbi.nlm.nih.gov | |
Description | Data deposited in or computed by PubChem | |
InChI Key |
USVNNHYNCCJCCP-UHFFFAOYSA-N | |
Source | PubChem | |
URL | https://pubchem.ncbi.nlm.nih.gov | |
Description | Data deposited in or computed by PubChem | |
Canonical SMILES |
C1=CC=C(C=C1)CC(=O)OC2=CC=CC=C2 | |
Source | PubChem | |
URL | https://pubchem.ncbi.nlm.nih.gov | |
Description | Data deposited in or computed by PubChem | |
Molecular Formula |
C14H12O2 | |
Source | PubChem | |
URL | https://pubchem.ncbi.nlm.nih.gov | |
Description | Data deposited in or computed by PubChem | |
DSSTOX Substance ID |
DTXSID40222527 | |
Record name | Phenyl phenylacetate | |
Source | EPA DSSTox | |
URL | https://comptox.epa.gov/dashboard/DTXSID40222527 | |
Description | DSSTox provides a high quality public chemistry resource for supporting improved predictive toxicology. | |
Molecular Weight |
212.24 g/mol | |
Source | PubChem | |
URL | https://pubchem.ncbi.nlm.nih.gov | |
Description | Data deposited in or computed by PubChem | |
CAS No. |
722-01-0 | |
Record name | Phenyl phenylacetate | |
Source | ChemIDplus | |
URL | https://pubchem.ncbi.nlm.nih.gov/substance/?source=chemidplus&sourceid=0000722010 | |
Description | ChemIDplus is a free, web search system that provides access to the structure and nomenclature authority files used for the identification of chemical substances cited in National Library of Medicine (NLM) databases, including the TOXNET system. | |
Record name | Phenyl phenylacetate | |
Source | EPA DSSTox | |
URL | https://comptox.epa.gov/dashboard/DTXSID40222527 | |
Description | DSSTox provides a high quality public chemistry resource for supporting improved predictive toxicology. | |
Synthesis routes and methods
Procedure details
Retrosynthesis Analysis
AI-Powered Synthesis Planning: Our tool employs the Template_relevance Pistachio, Template_relevance Bkms_metabolic, Template_relevance Pistachio_ringbreaker, Template_relevance Reaxys, Template_relevance Reaxys_biocatalysis model, leveraging a vast database of chemical reactions to predict feasible synthetic routes.
One-Step Synthesis Focus: Specifically designed for one-step synthesis, it provides concise and direct routes for your target compounds, streamlining the synthesis process.
Accurate Predictions: Utilizing the extensive PISTACHIO, BKMS_METABOLIC, PISTACHIO_RINGBREAKER, REAXYS, REAXYS_BIOCATALYSIS database, our tool offers high-accuracy predictions, reflecting the latest in chemical research and data.
Strategy Settings
Precursor scoring | Relevance Heuristic |
---|---|
Min. plausibility | 0.01 |
Model | Template_relevance |
Template Set | Pistachio/Bkms_metabolic/Pistachio_ringbreaker/Reaxys/Reaxys_biocatalysis |
Top-N result to add to graph | 6 |
Feasible Synthetic Routes
Disclaimer and Information on In-Vitro Research Products
Please be aware that all articles and product information presented on BenchChem are intended solely for informational purposes. The products available for purchase on BenchChem are specifically designed for in-vitro studies, which are conducted outside of living organisms. In-vitro studies, derived from the Latin term "in glass," involve experiments performed in controlled laboratory settings using cells or tissues. It is important to note that these products are not categorized as medicines or drugs, and they have not received approval from the FDA for the prevention, treatment, or cure of any medical condition, ailment, or disease. We must emphasize that any form of bodily introduction of these products into humans or animals is strictly prohibited by law. It is essential to adhere to these guidelines to ensure compliance with legal and ethical standards in research and experimentation.