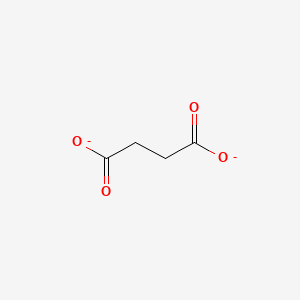
Succinate
Overview
Description
Succinate (C₄H₆O₄), also known as succinic acid in its protonated form, is a dicarboxylic acid integral to the tricarboxylic acid (TCA) cycle. It serves as a key metabolic intermediate, contributing to ATP synthesis via oxidative phosphorylation and acting as a signaling molecule in hypoxia responses by stabilizing hypoxia-inducible factor 1α (HIF-1α) . Industrially, this compound is a precursor for biodegradable polymers, pharmaceuticals, and food additives, owing to its dual functionality (two carboxylic acid groups) and reactivity .
Preparation Methods
Chemical Synthesis Methods
Acid-Catalyzed Esterification and Salt-Mediated Separation
The patent CN109053442A outlines a method for preparing succinate esters via reaction of succinic acid with alcohols in the presence of magnesium chloride (MgCl₂), calcium chloride (CaCl₂), or zinc chloride (ZnCl₂) at 50–100°C . These hydrochlorides act as catalysts and phase-separation enhancers, enabling yields above 90% for diesters like dibutyl this compound. The process involves:
-
Dissolving succinic acid in an aqueous solution containing ≥5 wt% MgCl₂.
-
Introducing alcohols (e.g., butanol) to form esters under mild conditions (30–130°C, 1–5 bar).
-
Separating the organic ester phase from the aqueous hydrochloride solution via liquid-liquid extraction .
Key Advantage : The hydrochloride catalyst remains recyclable, reducing waste. For instance, MgCl₂ can be thermally decomposed at 300°C to regenerate magnesia (MgO) and hydrogen chloride (HCl), which are reused in fermentation neutralization .
Petrochemical Routes and Their Limitations
Traditional methods convert maleic anhydride to this compound through hydrogenation, but these rely on non-renewable feedstocks and generate high CO₂ emissions . A comparative analysis reveals biological routes now surpass petrochemical methods in sustainability:
Parameter | Petrochemical Route | Biological Route |
---|---|---|
Feedstock | Maleic anhydride | Glucose/Glycerol |
Yield (g/g) | 0.65 | 0.75–0.85 |
CO₂ Emissions (kg/kg product) | 2.1 | 0.3 |
Energy Consumption (MJ/kg) | 45 | 18 |
Biological Production Methods
Anaerobic Fermentation in Escherichia coli
Engineered E. coli strains (e.g., AFP111, KJ060) divert carbon flux toward this compound by inactivating competing pathways (e.g., lactate dehydrogenase, ldhA) and overexpressing anaplerotic enzymes like phosphoenolpyruvate carboxylase (ppc) . Under anaerobic conditions with CO₂ sparging:
-
Glucose is converted to phosphoenolpyruvate (PEP), which fixes CO₂ to form oxaloacetate.
-
Reductive tricarboxylic acid (TCA) cycle enzymes reduce oxaloacetate to this compound via malate and fumarate .
Strain AFP184 achieves a yield of 0.50 g this compound/g xylose using lignocellulosic hydrolysates, demonstrating adaptability to diverse feedstocks .
Aerobic Production and Metabolic Balancing
Aerobic processes, though less common, utilize oxidative TCA cycle flux with minimal byproduct formation. Overexpression of pyruvate carboxylase (pyc) in E. coli redirects pyruvate to oxaloacetate, boosting this compound titer to 58 g/L in fed-batch reactors .
Industrial Fermentation Processes
Integrated Biorefinery Approaches
The patent CN109053442A describes an integrated system coupling fermentation, acidification, and esterification :
-
Fermentation : Actinobacillus succinogenes produces Magnesium this compound from glucose.
-
Acidification : HCl reacts with Magnesium this compound to release succinic acid.
-
Esterification : Succinic acid reacts with butanol in MgCl₂ solution to form dibutyl this compound.
This闭环 process recycles MgCl₂ and minimizes purification steps, reducing costs by 30% compared to standalone fermentation .
Feedstock Flexibility and Waste Valorization
Recent studies highlight glycerol, a biodiesel byproduct, as a low-cost substrate. E. coli strain XZ721 produces 102 mM this compound from 128 mM glycerol (0.8 mol/mol yield) under anaerobic conditions . Agricultural residues like wheat straw and corn stover are also viable, with Fibrobacter succinogenes achieving 2.0 g/L this compound from pretreated biomass .
Recent Advances and Innovations
Systems Biology and CRISPR-Based Engineering
Genome-scale models (GEMs) predict optimal gene knockout targets for maximizing this compound yield. For example, in silico simulations identified sdhAB (this compound dehydrogenase) deletion as critical for reducing this compound degradation . CRISPR interference (CRISPRi) has dynamically regulated competing pathways in Corynebacterium glutamicum, increasing titers by 40% .
Novel Separation Techniques
Temperature-responsive ionic liquids (TRILs) selectively extract this compound from fermentation broths at 40°C, achieving 95% recovery efficiency. This method reduces energy use by 50% compared to conventional distillation .
Comparative Analysis of Preparation Methods
Method | Yield (g/g) | Cost ($/kg) | Scalability | Environmental Impact |
---|---|---|---|---|
Chemical (Maleic Anhydride) | 0.65 | 1.20 | High | High CO₂ emissions |
Anaerobic Fermentation | 0.75 | 0.90 | Moderate | Low waste generation |
Aerobic Fermentation | 0.68 | 1.10 | Low | Moderate energy use |
Integrated Biorefinery | 0.82 | 0.75 | High | Closed-loop system |
Chemical Reactions Analysis
Types of Reactions: Succinate undergoes various chemical reactions, including:
Common Reagents and Conditions:
Oxidation: Common reagents include molecular oxygen and enzymes such as this compound dehydrogenase.
Reduction: Reducing agents like sodium borohydride or biological enzymes.
Substitution: Alcohols and acid catalysts are typically used in esterification reactions.
Major Products:
Oxidation: Fumarate
Reduction: Succinic semialdehyde, gamma-aminobutyric acid
Substitution: Succinic esters
Scientific Research Applications
Industrial Applications
1. Chemical Production
Succinate is recognized as a key building block in the production of various chemicals. It serves as a precursor for:
- Butanediol : Used in the manufacture of plastics and solvents.
- Tetrahydrofuran : A solvent for polymers and coatings.
- Pyrrolidone : Utilized in pharmaceuticals and personal care products.
In 2001, approximately 10 million pounds of this compound were produced from petrochemicals, with efforts ongoing to shift production to more sustainable methods such as fermentation from biomass sources like corn and sorghum .
Chemical Derived from this compound | Application |
---|---|
Butanediol | Plastics, solvents |
Tetrahydrofuran | Coatings, adhesives |
Pyrrolidone | Pharmaceuticals, cosmetics |
2. Food Industry
This compound is employed as a flavor enhancer in food products. Its ability to enhance taste profiles makes it valuable in the formulation of various processed foods .
3. Biopolymers
Recent studies have explored the incorporation of this compound into biopolymers. For example, poly(ethylene this compound-co-lactic acid) has been developed as a multifunctional additive, enhancing mechanical properties while promoting biodegradability .
Biological Applications
1. Metabolic Supplementation
Research indicates that this compound supplementation can improve mitochondrial function and metabolic performance in various biological systems. In studies involving traumatic brain injury (TBI) patients, this compound was shown to enhance glial oxidative metabolism, leading to improved energy state and reduced lactate levels .
- Case Study : A clinical trial demonstrated that this compound administration resulted in a significant decrease in the lactate-to-pyruvate ratio, indicating enhanced mitochondrial activity .
Parameter | Control Group | This compound Group |
---|---|---|
Lactate/Pyruvate Ratio | Higher | Lower |
Pyruvate Levels | Lower | Higher |
2. Therapeutic Potential
This compound's role in inflammatory signaling has garnered attention for its potential therapeutic applications. It has been implicated in enhancing immune responses and could serve as a target for developing treatments for conditions characterized by inflammation .
- Therapeutic Use Cases :
- Neurodegenerative diseases
- Mitochondrial dysfunction
- Inflammatory disorders
Computational Methods in this compound Production
The application of computational methods has been instrumental in optimizing this compound production processes. Studies have highlighted the integration of theoretical approaches with experimental techniques to enhance yields through metabolic engineering .
Year | Studies Conducted | Successful Implementations |
---|---|---|
2002-2016 | 26 | 10 |
This approach has led to innovative strategies for producing this compound more efficiently, emphasizing the importance of combining computational analysis with practical applications.
Mechanism of Action
Succinate exerts its effects primarily through its role in the tricarboxylic acid cycle. It serves as an electron donor in the production of fumaric acid and flavin adenine dinucleotide (FADH2), which are essential for cellular respiration and energy production . Additionally, this compound acts as a signaling molecule, influencing various cellular processes such as gene expression and immune responses .
Comparison with Similar Compounds
Dicarboxylates in Metabolic and Transport Contexts
Succinate belongs to the dicarboxylate family, which includes compounds like fumarate, malate, and α-ketoglutarate. These share structural similarities but differ in transporter affinities and metabolic roles:
- Transport Selectivity : Rat NaDC3 transporter preferentially binds this compound, fumarate, and β-ketoglutarate over tricarboxylates (citrate, isocitrate) due to steric and charge compatibility .
- Metabolic Flux: Unlike citrate (a tricarboxylate), this compound is a central node in the TCA cycle, linking carbohydrate metabolism to oxidative phosphorylation. Fumarate, a structural isomer, participates in the urea cycle and redox balance .
Table 1: Dicarboxylate Comparison
Compound | Transporter Affinity (NaDC3) | Metabolic Role |
---|---|---|
This compound | High | TCA cycle, HIF-1α stabilization |
Fumarate | Moderate | Urea cycle, redox mediator |
Citrate | Low | Fatty acid synthesis, glycolysis regulation |
Esters and Salts: Functional Derivatives
This compound esters and salts are widely used in pharmaceuticals and food science:
- Alpha-Tocopheryl this compound (TS) and Cholesteryl this compound (CS): These esters exhibit antitumor activity by inhibiting leukemia cell proliferation as intact molecules, unlike hydrolyzed derivatives (e.g., free alpha-tocopherol or cholesterol). TS and CS also protect normal bone marrow cells from doxorubicin toxicity .
- Octenyl this compound Starch : Enhances rheological properties in food matrices, improving gelatinization and retrogradation resistance compared to native starch .
- Sodium Sulfothis compound : A surfactant with applications in pharmaceuticals (e.g., docusate sodium) due to its emulsifying properties, contrasting with simpler salts like disodium this compound used in buffering .
Table 2: Key this compound Derivatives
Derivative | Application | Unique Property |
---|---|---|
Alpha-Tocopheryl this compound | Anticancer therapy | Intact compound activity, selective cytotoxicity |
Octenyl this compound Starch | Food encapsulation | Improved viscosity and stability |
Sodium Sulfothis compound | Pharmaceuticals (laxatives) | Surfactant action, low toxicity |
Polybutylene this compound (PBS): A Biodegradable Polymer
PBS, synthesized from succinic acid and 1,4-butanediol, is compared to fossil-based polymers (PET, PP) and biopolymers (PLA):
- Mechanical Properties : PBS exhibits tensile strength (∼30 MPa) and elongation (∼300%) comparable to polyethylene (PE) but lower than PET .
- Biodegradability: PBS degrades via ester bond hydrolysis, unlike non-biodegradable PET. However, it has higher light permeability and lower water vapor resistance than PLA .
- Production : Bio-based PBS uses microbial fermentation of glucose, reducing reliance on fossil feedstocks .
Table 3: Polymer Comparison
Polymer | Tensile Strength (MPa) | Biodegradability | Key Limitation |
---|---|---|---|
PBS | 30–35 | Yes | High cost, low UV resistance |
PET | 50–75 | No | Non-biodegradable |
PLA | 45–70 | Yes | Brittleness |
Microbial Production and Fermentation
Anaerobiospirillum succiniciproducens produces this compound under high CO₂ conditions, yielding 10–15 g/L, outperforming lactate fermentation in ATP efficiency. This contrasts with Escherichia coli, which requires genetic engineering for this compound overproduction .
Biological Activity
Succinate, a key intermediate in the tricarboxylic acid (TCA) cycle, has garnered significant attention for its diverse biological activities beyond its traditional role in cellular metabolism. Recent studies have elucidated its involvement in various physiological and pathological processes, particularly in inflammation, immune regulation, and cancer progression. This article aims to provide a comprehensive overview of the biological activity of this compound, supported by data tables, case studies, and detailed research findings.
Overview of this compound
This compound is a four-carbon dicarboxylic acid that plays a crucial role in the TCA cycle within mitochondria. It is produced during the conversion of succinyl-CoA to this compound by succinyl-CoA synthetase and is subsequently oxidized to fumarate by this compound dehydrogenase (SDH). Under conditions of hypoxia or metabolic stress, this compound can accumulate and exert various signaling effects through its receptor, this compound receptor 1 (SUCNR1), a G protein-coupled receptor (GPCR) implicated in numerous biological functions.
1. Inflammatory Response Modulation
This compound has been shown to play dual roles in inflammation, acting as both a pro-inflammatory and anti-inflammatory mediator depending on the context:
- Pro-inflammatory Effects : Elevated levels of this compound can enhance the production of reactive oxygen species (ROS) and stabilize hypoxia-inducible factor 1-alpha (HIF-1α), leading to increased expression of pro-inflammatory cytokines. This has been observed in macrophages activated by lipopolysaccharide (LPS), where this compound promotes reverse electron transport in mitochondria, resulting in heightened ROS production and inflammatory signaling .
- Anti-inflammatory Effects : Conversely, recent studies suggest that SUCNR1 activation may also mediate anti-inflammatory responses under certain conditions. The precise mechanisms remain an area of active investigation .
2. Role in Immune Regulation
This compound influences various immune cell functions:
- Dendritic Cells : It enhances the migration and activation of dendritic cells, which are critical for initiating adaptive immune responses. This effect is mediated through SUCNR1 signaling .
- Macrophages : In macrophages, this compound modulates cytokine production and influences metabolic adaptations necessary for effective immune responses .
3. Epigenetic Modifications
This compound has been implicated in epigenetic regulation through its ability to inhibit histone demethylases, leading to increased DNA methylation and alterations in gene expression. This post-translational modification can significantly impact cellular functions and has been linked to various diseases, including cancer .
Table 1: Summary of Research Findings on this compound's Biological Activity
Study | Focus Area | Key Findings |
---|---|---|
Rubic et al., 2008 | Immune Activation | This compound enhances platelet aggregation and activates immune cells via SUCNR1. |
Mills et al., 2014 | Inflammation | Demonstrated that this compound stabilizes HIF-1α and promotes pro-inflammatory cytokine production in macrophages. |
Dalla Pozza et al., 2020 | Cancer | This compound inhibits tumor growth by modulating immune cell function and enhancing anti-tumor immunity. |
Peace & O'Neill, 2022 | Immune Cell Migration | This compound promotes migration of monocyte-derived dendritic cells via SUCNR1 activation. |
Implications for Therapeutic Targeting
Given its multifaceted roles in inflammation and immunity, targeting this compound metabolism presents a promising therapeutic strategy for various diseases:
- Chronic Inflammatory Diseases : Modulating this compound levels could help control inflammatory responses in conditions such as rheumatoid arthritis or inflammatory bowel disease.
- Cancer Therapy : By manipulating this compound signaling pathways, it may be possible to enhance anti-tumor immunity or inhibit tumor progression .
Q & A
Q. How can researchers design experiments to distinguish between succinate’s roles in mitochondrial electron transport versus its signaling functions in metabolic pathways?
Methodological Answer:
To isolate this compound’s dual roles, employ compartment-specific inhibitors (e.g., malonate for mitochondrial Complex II) alongside genetic knockouts of this compound receptors (e.g., SUCNR1). Use stable isotope tracing (e.g., ¹³C-labeled this compound) to track metabolic flux in mitochondrial vs. cytosolic pathways. Pair this with phosphoproteomics to identify signaling cascades activated by extracellular this compound . For rigor, include controls with this compound dehydrogenase (SDH)-deficient cell lines to rule out mitochondrial confounding effects .
Q. What statistical approaches are optimal for modeling the relationship between reaction parameters and this compound yield in enzymatic synthesis?
Methodological Answer:
Response Surface Methodology (RSM) with Central Composite Design (CCD) is widely validated for optimizing enzymatic this compound production. For example, in lipase-catalyzed succinic acid ester synthesis, CCD can model interactions between variables (e.g., temperature, pH, enzyme loading) and predict optimal conditions . Use ANOVA to rank parameter significance (e.g., solution concentration as a dominant factor in polybutylene this compound nanofiber production) and validate models via confirmation experiments .
Q. How should contradictory data on this compound’s pro- versus anti-inflammatory effects be reconciled in systematic reviews?
Methodological Answer:
Apply PICOT framework (Population, Intervention, Comparison, Outcome, Time) to standardize inclusion criteria. For example:
- Population: Macrophages vs. epithelial cells.
- Intervention: this compound concentration gradients (0.1–10 mM).
- Outcome: IL-1β secretion vs. HIF-1α stabilization.
Use meta-regression to assess dose-response relationships and cell-type heterogeneity. Ensure methodological quality appraisal via tools like COSMOS-E to exclude studies with poor SDH activity validation .
Q. What techniques validate the structural integrity of this compound-containing polymers (e.g., PBS) under varying fabrication conditions?
Methodological Answer:
Combine scanning electron microscopy (SEM) for nanofiber morphology with differential scanning calorimetry (DSC) to assess thermal stability (e.g., melting points of PBS). For chemical stability, use FTIR spectroscopy to monitor ester bond integrity. Employ full factorial experimental designs to test interactions between parameters (e.g., air pressure, feed rate) and material properties (e.g., contact angle, permeability) .
Q. How can this compound’s extracellular accumulation be quantified in real-time during cell culture experiments?
Methodological Answer:
Implement microfluidic biosensors or LC-MS/MS for high-temporal-resolution measurements. For dynamic profiling, use enzyme-linked assays (e.g., this compound oxidase-based kits) with minimal cell disruption. Normalize data to cell density (OD₆₀₀) and validate via intracellular vs. extracellular this compound compartmentalization assays .
Q. What are best practices for reporting this compound-related findings to ensure reproducibility in synthetic biology studies?
Methodological Answer:
- Materials and Methods : Specify this compound source (e.g., Sigma-Aldridch, ≥99% purity), strain background (e.g., E. coli BW25113 ΔsucA), and growth media composition.
- Data Reporting : Include raw data tables for metabolite concentrations and statistical parameters (e.g., R² values for regression models).
- Supplemental Information : Provide NMR spectra for this compound derivative verification and CRISPR-editing primers .
Q. How do researchers address confounding variables when studying this compound’s role in cancer metabolism?
Methodological Answer:
Use isogenic cell lines (e.g., wild-type vs. SDH-mutant) to isolate this compound’s effects. Apply stable isotope-resolved metabolomics (SIRM) with ¹³C-glutamine to differentiate reductive carboxylation from oxidative TCA cycle activity. Control for hypoxia via O₂-controlled bioreactors to decouple HIF-1α effects from this compound accumulation .
Q. What frameworks guide the formulation of mechanistic hypotheses about this compound-driven epigenetic modifications?
Methodological Answer:
Leverage multi-omics integration :
DNA methylome profiling (e.g., WGBS) to identify hyper/hypomethylated regions.
Chromatin immunoprecipitation (ChIP) for histone modification analysis (e.g., H3K4me3).
Correlation with transcriptomics (RNA-seq) to link this compound levels to gene expression. Validate via This compound analogue treatments (e.g., dimethyl this compound) in in vitro models .
Q. How can contradictory findings about this compound’s antioxidant vs. pro-oxidant effects be resolved experimentally?
Methodological Answer:
Design dose- and time-dependent assays:
- ROS quantification : Use DCFDA for general ROS and MitoSOX for mitochondrial superoxide.
- Enzyme activity assays : Measure SOD and catalase activity alongside this compound levels.
- Genetic validation : Knock down Nrf2 or NOX4 to test pathway-specific effects. Statistical analysis via two-way ANOVA can isolate interaction effects between this compound concentration and oxidative stress markers .
Q. What methodologies ensure robust structural characterization of novel this compound derivatives?
Methodological Answer:
For novel esters or metal-succinate complexes:
X-ray crystallography for unambiguous structural confirmation.
High-resolution mass spectrometry (HRMS) to verify molecular formulae.
Dynamic light scattering (DLS) for aggregation state analysis in solution.
Include control experiments with unmodified this compound to benchmark spectral data (e.g., ¹H NMR δ 2.40–2.60 ppm) .
Properties
IUPAC Name |
butanedioate | |
---|---|---|
Source | PubChem | |
URL | https://pubchem.ncbi.nlm.nih.gov | |
Description | Data deposited in or computed by PubChem | |
InChI |
InChI=1S/C4H6O4/c5-3(6)1-2-4(7)8/h1-2H2,(H,5,6)(H,7,8)/p-2 | |
Source | PubChem | |
URL | https://pubchem.ncbi.nlm.nih.gov | |
Description | Data deposited in or computed by PubChem | |
InChI Key |
KDYFGRWQOYBRFD-UHFFFAOYSA-L | |
Source | PubChem | |
URL | https://pubchem.ncbi.nlm.nih.gov | |
Description | Data deposited in or computed by PubChem | |
Canonical SMILES |
C(CC(=O)[O-])C(=O)[O-] | |
Source | PubChem | |
URL | https://pubchem.ncbi.nlm.nih.gov | |
Description | Data deposited in or computed by PubChem | |
Molecular Formula |
C4H4O4-2 | |
Source | PubChem | |
URL | https://pubchem.ncbi.nlm.nih.gov | |
Description | Data deposited in or computed by PubChem | |
DSSTOX Substance ID |
DTXSID4036772 | |
Record name | Succinate | |
Source | EPA DSSTox | |
URL | https://comptox.epa.gov/dashboard/DTXSID4036772 | |
Description | DSSTox provides a high quality public chemistry resource for supporting improved predictive toxicology. | |
Molecular Weight |
116.07 g/mol | |
Source | PubChem | |
URL | https://pubchem.ncbi.nlm.nih.gov | |
Description | Data deposited in or computed by PubChem | |
CAS No. |
56-14-4 | |
Record name | Succinate | |
Source | CAS Common Chemistry | |
URL | https://commonchemistry.cas.org/detail?cas_rn=56-14-4 | |
Description | CAS Common Chemistry is an open community resource for accessing chemical information. Nearly 500,000 chemical substances from CAS REGISTRY cover areas of community interest, including common and frequently regulated chemicals, and those relevant to high school and undergraduate chemistry classes. This chemical information, curated by our expert scientists, is provided in alignment with our mission as a division of the American Chemical Society. | |
Explanation | The data from CAS Common Chemistry is provided under a CC-BY-NC 4.0 license, unless otherwise stated. | |
Record name | Succinate dianion | |
Source | ChemIDplus | |
URL | https://pubchem.ncbi.nlm.nih.gov/substance/?source=chemidplus&sourceid=0000056144 | |
Description | ChemIDplus is a free, web search system that provides access to the structure and nomenclature authority files used for the identification of chemical substances cited in National Library of Medicine (NLM) databases, including the TOXNET system. | |
Record name | Succinate | |
Source | EPA DSSTox | |
URL | https://comptox.epa.gov/dashboard/DTXSID4036772 | |
Description | DSSTox provides a high quality public chemistry resource for supporting improved predictive toxicology. | |
Record name | Butanedioic acid, ion(2-) | |
Source | European Chemicals Agency (ECHA) | |
URL | https://echa.europa.eu/information-on-chemicals | |
Description | The European Chemicals Agency (ECHA) is an agency of the European Union which is the driving force among regulatory authorities in implementing the EU's groundbreaking chemicals legislation for the benefit of human health and the environment as well as for innovation and competitiveness. | |
Explanation | Use of the information, documents and data from the ECHA website is subject to the terms and conditions of this Legal Notice, and subject to other binding limitations provided for under applicable law, the information, documents and data made available on the ECHA website may be reproduced, distributed and/or used, totally or in part, for non-commercial purposes provided that ECHA is acknowledged as the source: "Source: European Chemicals Agency, http://echa.europa.eu/". Such acknowledgement must be included in each copy of the material. ECHA permits and encourages organisations and individuals to create links to the ECHA website under the following cumulative conditions: Links can only be made to webpages that provide a link to the Legal Notice page. | |
Record name | SUCCINATE DIANION | |
Source | FDA Global Substance Registration System (GSRS) | |
URL | https://gsrs.ncats.nih.gov/ginas/app/beta/substances/AJ326AG789 | |
Description | The FDA Global Substance Registration System (GSRS) enables the efficient and accurate exchange of information on what substances are in regulated products. Instead of relying on names, which vary across regulatory domains, countries, and regions, the GSRS knowledge base makes it possible for substances to be defined by standardized, scientific descriptions. | |
Explanation | Unless otherwise noted, the contents of the FDA website (www.fda.gov), both text and graphics, are not copyrighted. They are in the public domain and may be republished, reprinted and otherwise used freely by anyone without the need to obtain permission from FDA. Credit to the U.S. Food and Drug Administration as the source is appreciated but not required. | |
Synthesis routes and methods I
Procedure details
Synthesis routes and methods II
Procedure details
Synthesis routes and methods III
Procedure details
Synthesis routes and methods IV
Procedure details
Synthesis routes and methods V
Procedure details
Retrosynthesis Analysis
AI-Powered Synthesis Planning: Our tool employs the Template_relevance Pistachio, Template_relevance Bkms_metabolic, Template_relevance Pistachio_ringbreaker, Template_relevance Reaxys, Template_relevance Reaxys_biocatalysis model, leveraging a vast database of chemical reactions to predict feasible synthetic routes.
One-Step Synthesis Focus: Specifically designed for one-step synthesis, it provides concise and direct routes for your target compounds, streamlining the synthesis process.
Accurate Predictions: Utilizing the extensive PISTACHIO, BKMS_METABOLIC, PISTACHIO_RINGBREAKER, REAXYS, REAXYS_BIOCATALYSIS database, our tool offers high-accuracy predictions, reflecting the latest in chemical research and data.
Strategy Settings
Precursor scoring | Relevance Heuristic |
---|---|
Min. plausibility | 0.01 |
Model | Template_relevance |
Template Set | Pistachio/Bkms_metabolic/Pistachio_ringbreaker/Reaxys/Reaxys_biocatalysis |
Top-N result to add to graph | 6 |
Feasible Synthetic Routes
Disclaimer and Information on In-Vitro Research Products
Please be aware that all articles and product information presented on BenchChem are intended solely for informational purposes. The products available for purchase on BenchChem are specifically designed for in-vitro studies, which are conducted outside of living organisms. In-vitro studies, derived from the Latin term "in glass," involve experiments performed in controlled laboratory settings using cells or tissues. It is important to note that these products are not categorized as medicines or drugs, and they have not received approval from the FDA for the prevention, treatment, or cure of any medical condition, ailment, or disease. We must emphasize that any form of bodily introduction of these products into humans or animals is strictly prohibited by law. It is essential to adhere to these guidelines to ensure compliance with legal and ethical standards in research and experimentation.