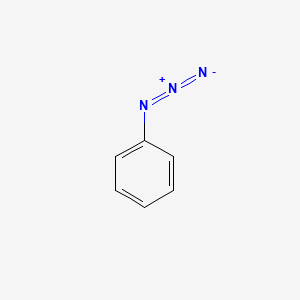
Azidobenzene
Overview
Description
Azidobenzene is an organic compound characterized by the presence of an azido group (-N₃) attached to a benzene ring. This compound is known for its versatility and reactivity, making it a valuable intermediate in organic synthesis and various chemical applications. This compound is a member of the broader class of azides, which are compounds containing the azido functional group.
Mechanism of Action
Target of Action
Azidobenzene, also known as Phenyl azide, is a versatile compound that has been used in various scientific fields . The primary targets of this compound are molecular structures that can undergo cis-trans isomerization . This property makes this compound an excellent candidate to function as a molecular switch .
Mode of Action
This compound interacts with its targets through a process known as photoisomerization . This process involves the conversion of this compound from its trans form to its cis form in the presence of appropriate radiation . This isomerization can be reversed, allowing this compound to switch between these two forms .
Biochemical Pathways
The photoisomerization of this compound affects the biochemical pathways related to the function of the target molecules. For instance, in the case of this compound-modified DNA, the trans-cis isomerization can lead to the destabilization of the DNA duplex configuration, eventually leading to DNA unzipping . This process can be reversed, allowing the DNA to return to its original configuration .
Pharmacokinetics
It’s important to note that the pharmacokinetic properties of a compound can significantly impact its bioavailability and efficacy .
Result of Action
The molecular and cellular effects of this compound’s action are primarily due to its ability to undergo photoisomerization. This property allows this compound to act as a molecular switch, altering the structure and function of target molecules . For example, this compound-modified DNA can switch between a hybridized (duplex) and dehybridized (unzipped) state .
Action Environment
The action, efficacy, and stability of this compound can be influenced by various environmental factors. For instance, the efficiency of the photoisomerization process can be affected by the wavelength and intensity of the radiation used . Additionally, the presence of certain ions can influence the half-life of the cis form of this compound .
Biochemical Analysis
Biochemical Properties
Azidobenzene plays a crucial role in biochemical reactions, particularly in the context of photoresponsive systems. It interacts with various enzymes, proteins, and other biomolecules through its azide group, which can form covalent bonds with nucleophiles such as amines and thiols. This interaction is often utilized in bioconjugation techniques to label or modify biomolecules. For instance, this compound can react with lysine residues on proteins, leading to the formation of stable amide bonds .
In addition to its covalent interactions, this compound can also engage in non-covalent interactions with biomolecules. Its aromatic structure allows it to participate in π-π stacking interactions with aromatic amino acids such as phenylalanine, tyrosine, and tryptophan. These interactions can influence the folding and stability of proteins, as well as their interactions with other biomolecules .
Cellular Effects
This compound has been shown to affect various types of cells and cellular processes. One of its most notable effects is its ability to modulate cell signaling pathways. Upon exposure to light, this compound undergoes photoisomerization, which can lead to changes in the conformation of proteins and other biomolecules it is attached to. This conformational change can activate or inhibit signaling pathways, depending on the specific context .
Furthermore, this compound can influence gene expression by interacting with transcription factors and other regulatory proteins. By modifying these proteins, this compound can alter their ability to bind to DNA and regulate the transcription of target genes. This can result in changes in cellular metabolism and other physiological processes .
Molecular Mechanism
The molecular mechanism of this compound’s action is primarily based on its ability to undergo photoisomerization. In its trans form, this compound is relatively stable and can interact with biomolecules through covalent and non-covalent interactions. Upon exposure to light, it isomerizes to its cis form, which has a different spatial arrangement of atoms. This change in conformation can disrupt existing interactions and create new ones, leading to changes in the activity and function of biomolecules .
This compound can also act as an enzyme inhibitor or activator, depending on the specific enzyme and the nature of the interaction. For example, it can inhibit enzymes by binding to their active sites and blocking substrate access. Alternatively, it can activate enzymes by inducing conformational changes that enhance their catalytic activity .
Temporal Effects in Laboratory Settings
In laboratory settings, the effects of this compound can change over time due to its stability and degradation. This compound is generally stable under dark conditions, but prolonged exposure to light can lead to its degradation and loss of activity. This degradation can result in the formation of byproducts that may have different biochemical properties and effects on cellular function .
Long-term studies have shown that this compound can have lasting effects on cellular function, particularly in the context of gene expression and cell signaling. These effects can persist even after the compound has been degraded, suggesting that this compound can induce long-term changes in cellular physiology .
Dosage Effects in Animal Models
The effects of this compound in animal models can vary with different dosages. At low doses, this compound can modulate cellular processes without causing significant toxicity. At high doses, it can induce toxic effects, including cell death and tissue damage. These toxic effects are often dose-dependent and can be influenced by factors such as the route of administration and the duration of exposure .
Threshold effects have also been observed, where a certain minimum dose of this compound is required to elicit a measurable biological response. This threshold can vary depending on the specific biological system and the nature of the interaction between this compound and biomolecules .
Metabolic Pathways
This compound is involved in various metabolic pathways, primarily through its interactions with enzymes and cofactors. It can be metabolized by enzymes such as cytochrome P450, which can oxidize the azide group to form reactive intermediates. These intermediates can then react with other biomolecules, leading to the formation of covalent adducts and other modifications .
This compound can also affect metabolic flux by altering the activity of key enzymes involved in metabolic pathways. For example, it can inhibit enzymes involved in glycolysis and the tricarboxylic acid cycle, leading to changes in metabolite levels and energy production .
Transport and Distribution
Within cells and tissues, this compound is transported and distributed through various mechanisms. It can be taken up by cells through passive diffusion or active transport, depending on its chemical properties and the presence of specific transporters. Once inside the cell, this compound can interact with binding proteins that facilitate its transport to specific cellular compartments .
This compound can also accumulate in certain tissues, particularly those with high levels of metabolic activity. This accumulation can enhance its effects on cellular function and increase the likelihood of toxic effects at high doses .
Subcellular Localization
The subcellular localization of this compound can influence its activity and function. It can be targeted to specific compartments or organelles through the use of targeting signals or post-translational modifications. For example, this compound can be directed to the nucleus by attaching a nuclear localization signal, where it can interact with transcription factors and other nuclear proteins .
In addition, this compound can be localized to the mitochondria, where it can affect mitochondrial function and energy production. This localization can be achieved through the use of mitochondrial targeting sequences or by modifying this compound to enhance its affinity for mitochondrial membranes .
Preparation Methods
Synthetic Routes and Reaction Conditions: Azidobenzene can be synthesized through several methods, with the most common being the diazotization of aniline followed by azidation. The process involves the following steps:
Diazotization: Aniline is treated with nitrous acid (generated in situ from sodium nitrite and hydrochloric acid) to form a diazonium salt.
Azidation: The diazonium salt is then reacted with sodium azide to yield this compound.
The reaction conditions typically involve maintaining a low temperature (0-5°C) to ensure the stability of the diazonium salt and to prevent side reactions.
Industrial Production Methods: In industrial settings, the production of this compound follows similar principles but is scaled up to accommodate larger quantities. The use of continuous flow reactors and automated systems helps in maintaining precise control over reaction conditions, ensuring high yields and purity of the final product.
Chemical Reactions Analysis
Azidobenzene undergoes various chemical reactions, including:
Oxidation:
- This compound can be oxidized to form nitroso and nitro derivatives. Common oxidizing agents include potassium permanganate and chromium trioxide.
Reduction:
- Reduction of this compound typically yields aniline. This can be achieved using reducing agents such as hydrogen gas in the presence of a palladium catalyst or sodium borohydride.
Substitution:
- This compound can participate in nucleophilic substitution reactions, where the azido group is replaced by other nucleophiles. For example, reaction with triphenylphosphine results in the formation of iminophosphorane.
Cycloaddition:
- This compound is known to undergo cycloaddition reactions, such as the Huisgen 1,3-dipolar cycloaddition, forming triazoles. This reaction is widely used in click chemistry.
Scientific Research Applications
Azidobenzene has a wide range of applications in scientific research, including:
Chemistry:
- This compound is used as a precursor in the synthesis of various heterocyclic compounds. Its reactivity makes it a valuable intermediate in organic synthesis.
Biology:
- In biological research, this compound derivatives are used as photoaffinity labels. These compounds can be activated by light to form covalent bonds with target biomolecules, aiding in the study of protein-ligand interactions.
Medicine:
- This compound derivatives have been explored for their potential use in drug delivery systems. The ability to undergo photoisomerization allows for controlled release of therapeutic agents.
Industry:
- This compound is used in the production of dyes and pigments. Its ability to form stable azo compounds makes it a valuable component in the textile and printing industries.
Comparison with Similar Compounds
Azidobenzene can be compared with other azido compounds, such as:
Phenyl azide: Similar to this compound, phenyl azide contains an azido group attached to a benzene ring. phenyl azide is less stable and more prone to decomposition.
Benzyl azide: This compound features an azido group attached to a benzyl group. Benzyl azide is more reactive than this compound and is commonly used in organic synthesis.
Azidopyridine: Azidopyridine contains an azido group attached to a pyridine ring. It exhibits different reactivity patterns compared to this compound due to the presence of the nitrogen atom in the ring.
This compound’s unique combination of stability and reactivity makes it a valuable compound in various chemical and biological applications. Its ability to undergo photoisomerization and participate in diverse chemical reactions sets it apart from other azido compounds.
Properties
IUPAC Name |
azidobenzene | |
---|---|---|
Source | PubChem | |
URL | https://pubchem.ncbi.nlm.nih.gov | |
Description | Data deposited in or computed by PubChem | |
InChI |
InChI=1S/C6H5N3/c7-9-8-6-4-2-1-3-5-6/h1-5H | |
Source | PubChem | |
URL | https://pubchem.ncbi.nlm.nih.gov | |
Description | Data deposited in or computed by PubChem | |
InChI Key |
CTRLRINCMYICJO-UHFFFAOYSA-N | |
Source | PubChem | |
URL | https://pubchem.ncbi.nlm.nih.gov | |
Description | Data deposited in or computed by PubChem | |
Canonical SMILES |
C1=CC=C(C=C1)N=[N+]=[N-] | |
Source | PubChem | |
URL | https://pubchem.ncbi.nlm.nih.gov | |
Description | Data deposited in or computed by PubChem | |
Molecular Formula |
C6H5N3 | |
Source | PubChem | |
URL | https://pubchem.ncbi.nlm.nih.gov | |
Description | Data deposited in or computed by PubChem | |
DSSTOX Substance ID |
DTXSID30878870 | |
Record name | Phenylazide | |
Source | EPA DSSTox | |
URL | https://comptox.epa.gov/dashboard/DTXSID30878870 | |
Description | DSSTox provides a high quality public chemistry resource for supporting improved predictive toxicology. | |
Molecular Weight |
119.12 g/mol | |
Source | PubChem | |
URL | https://pubchem.ncbi.nlm.nih.gov | |
Description | Data deposited in or computed by PubChem | |
CAS No. |
622-37-7 | |
Record name | Azidobenzene | |
Source | CAS Common Chemistry | |
URL | https://commonchemistry.cas.org/detail?cas_rn=622-37-7 | |
Description | CAS Common Chemistry is an open community resource for accessing chemical information. Nearly 500,000 chemical substances from CAS REGISTRY cover areas of community interest, including common and frequently regulated chemicals, and those relevant to high school and undergraduate chemistry classes. This chemical information, curated by our expert scientists, is provided in alignment with our mission as a division of the American Chemical Society. | |
Explanation | The data from CAS Common Chemistry is provided under a CC-BY-NC 4.0 license, unless otherwise stated. | |
Record name | Phenylazide | |
Source | ChemIDplus | |
URL | https://pubchem.ncbi.nlm.nih.gov/substance/?source=chemidplus&sourceid=0000622377 | |
Description | ChemIDplus is a free, web search system that provides access to the structure and nomenclature authority files used for the identification of chemical substances cited in National Library of Medicine (NLM) databases, including the TOXNET system. | |
Record name | Azidobenzene | |
Source | DTP/NCI | |
URL | https://dtp.cancer.gov/dtpstandard/servlet/dwindex?searchtype=NSC&outputformat=html&searchlist=512574 | |
Description | The NCI Development Therapeutics Program (DTP) provides services and resources to the academic and private-sector research communities worldwide to facilitate the discovery and development of new cancer therapeutic agents. | |
Explanation | Unless otherwise indicated, all text within NCI products is free of copyright and may be reused without our permission. Credit the National Cancer Institute as the source. | |
Record name | Phenylazide | |
Source | EPA DSSTox | |
URL | https://comptox.epa.gov/dashboard/DTXSID30878870 | |
Description | DSSTox provides a high quality public chemistry resource for supporting improved predictive toxicology. | |
Record name | Azidobenzene | |
Source | European Chemicals Agency (ECHA) | |
URL | https://echa.europa.eu/substance-information/-/substanceinfo/100.009.756 | |
Description | The European Chemicals Agency (ECHA) is an agency of the European Union which is the driving force among regulatory authorities in implementing the EU's groundbreaking chemicals legislation for the benefit of human health and the environment as well as for innovation and competitiveness. | |
Explanation | Use of the information, documents and data from the ECHA website is subject to the terms and conditions of this Legal Notice, and subject to other binding limitations provided for under applicable law, the information, documents and data made available on the ECHA website may be reproduced, distributed and/or used, totally or in part, for non-commercial purposes provided that ECHA is acknowledged as the source: "Source: European Chemicals Agency, http://echa.europa.eu/". Such acknowledgement must be included in each copy of the material. ECHA permits and encourages organisations and individuals to create links to the ECHA website under the following cumulative conditions: Links can only be made to webpages that provide a link to the Legal Notice page. | |
Record name | PHENYL AZIDE | |
Source | FDA Global Substance Registration System (GSRS) | |
URL | https://gsrs.ncats.nih.gov/ginas/app/beta/substances/2G0EH7N6YB | |
Description | The FDA Global Substance Registration System (GSRS) enables the efficient and accurate exchange of information on what substances are in regulated products. Instead of relying on names, which vary across regulatory domains, countries, and regions, the GSRS knowledge base makes it possible for substances to be defined by standardized, scientific descriptions. | |
Explanation | Unless otherwise noted, the contents of the FDA website (www.fda.gov), both text and graphics, are not copyrighted. They are in the public domain and may be republished, reprinted and otherwise used freely by anyone without the need to obtain permission from FDA. Credit to the U.S. Food and Drug Administration as the source is appreciated but not required. | |
Synthesis routes and methods I
Procedure details
Synthesis routes and methods II
Procedure details
Synthesis routes and methods III
Procedure details
Synthesis routes and methods IV
Procedure details
Retrosynthesis Analysis
AI-Powered Synthesis Planning: Our tool employs the Template_relevance Pistachio, Template_relevance Bkms_metabolic, Template_relevance Pistachio_ringbreaker, Template_relevance Reaxys, Template_relevance Reaxys_biocatalysis model, leveraging a vast database of chemical reactions to predict feasible synthetic routes.
One-Step Synthesis Focus: Specifically designed for one-step synthesis, it provides concise and direct routes for your target compounds, streamlining the synthesis process.
Accurate Predictions: Utilizing the extensive PISTACHIO, BKMS_METABOLIC, PISTACHIO_RINGBREAKER, REAXYS, REAXYS_BIOCATALYSIS database, our tool offers high-accuracy predictions, reflecting the latest in chemical research and data.
Strategy Settings
Precursor scoring | Relevance Heuristic |
---|---|
Min. plausibility | 0.01 |
Model | Template_relevance |
Template Set | Pistachio/Bkms_metabolic/Pistachio_ringbreaker/Reaxys/Reaxys_biocatalysis |
Top-N result to add to graph | 6 |
Feasible Synthetic Routes
Q1: What is the molecular formula and weight of azidobenzene?
A1: The molecular formula of this compound is C6H5N3, and its molecular weight is 119.12 g/mol.
Q2: What spectroscopic data is available for this compound?
A2: Researchers have extensively studied the spectroscopic properties of this compound using various techniques. Notably, its electronic absorption spectra and electronic structures have been investigated, along with those of related compounds like nitrenobenzene and benzenediazonium ion []. Furthermore, 13C NMR spectroscopy has been instrumental in studying the azide-tetrazole tautomerism in this compound and related heterocycles [].
Q3: What factors influence the thermal decomposition rate of this compound?
A4: The rate of this compound thermolysis is affected by several factors, including the presence and nature of substituents on the benzene ring. Electron-donating groups, especially those with a +R effect, can substantially enhance the decomposition rate []. Studies have also explored the impact of neighboring groups, such as carbonyl groups in locked conformations, on the thermolysis rates [, ]. Solvent effects and azide concentration further influence the kinetics and product distribution during thermal decomposition [].
Q4: Can this compound participate in click chemistry reactions?
A5: Yes, this compound is a valuable reagent in click chemistry, particularly in copper(I)-catalyzed azide-alkyne cycloaddition (CuAAC) reactions. It readily reacts with alkynes to form 1,2,3-triazole rings, enabling the synthesis of diversely functionalized molecules [, ]. This reaction has found applications in various fields, including the development of new sensors [].
Q5: How does the presence of Lewis acid catalysts affect the reactivity of this compound in cycloaddition reactions?
A6: Theoretical studies employing density functional theory (DFT) have revealed that Lewis acid catalysts, such as BF3, BCl3, and BBr3, can significantly influence both the kinetics and regioselectivity of 1,3-dipolar cycloaddition reactions involving this compound []. The presence of Lewis acids enhances the electrophilicity of the dipolarophile (e.g., acrolein), thereby lowering the reaction barrier and promoting regioselectivity.
Q6: Have computational methods been used to study this compound?
A7: Yes, computational chemistry, particularly DFT calculations, has been employed to investigate various aspects of this compound chemistry. For instance, DFT studies have been used to elucidate the mechanism of base-catalyzed cycloaddition reactions between this compound and alkynes []. Moreover, molecular docking simulations have provided insights into the regiospecificity and mechanism of [3+2] cycloaddition reactions of this compound with dipolarophiles like propiolaldehyde [].
Q7: How do substituents on the benzene ring affect the reactivity of this compound?
A8: The nature and position of substituents on the benzene ring can dramatically influence the reactivity of this compound. Electron-donating groups generally enhance the rate of thermal decomposition, with ortho-substituents exhibiting a more pronounced effect than para-substituents []. Conversely, electron-withdrawing groups tend to decrease the reactivity. These effects are attributed to the stabilization or destabilization of the transition state during azide decomposition.
Q8: Are there specific structural features that influence the neighboring group participation in this compound thermolysis?
A9: Yes, the effectiveness of neighboring group participation in this compound thermolysis is highly dependent on the nature and orientation of the neighboring group. For instance, studies on the pyrolysis of ortho-substituted azidobenzenes have revealed that groups like phenylazo, benzoyl, and formyl exhibit varying degrees of rate enhancement []. Notably, the "locked conformation" of carbonyl groups in specific this compound derivatives leads to substantially larger rate enhancements compared to freely rotating ortho substituents []. These observations highlight the importance of conformational factors and electronic effects in governing neighboring group participation.
Q9: How can the stability of this compound be improved?
A10: The stability of this compound can be enhanced by converting it into more stable derivatives or by incorporating it into specific formulations. For example, the formation of polycationically substituted azidobenzenes leads to increased stability against heat and shock []. Additionally, immobilizing this compound onto solid supports, such as polycarbonate, has been shown to enhance its stability and reusability, particularly in applications involving enzyme immobilization [].
Disclaimer and Information on In-Vitro Research Products
Please be aware that all articles and product information presented on BenchChem are intended solely for informational purposes. The products available for purchase on BenchChem are specifically designed for in-vitro studies, which are conducted outside of living organisms. In-vitro studies, derived from the Latin term "in glass," involve experiments performed in controlled laboratory settings using cells or tissues. It is important to note that these products are not categorized as medicines or drugs, and they have not received approval from the FDA for the prevention, treatment, or cure of any medical condition, ailment, or disease. We must emphasize that any form of bodily introduction of these products into humans or animals is strictly prohibited by law. It is essential to adhere to these guidelines to ensure compliance with legal and ethical standards in research and experimentation.