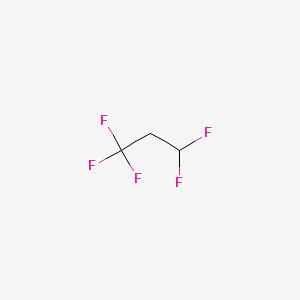
1,1,1,3,3-Pentafluoropropane
Overview
Description
1,1,1,3,3-Pentafluoropropane (HFC-245fa), with the molecular formula C₃H₃F₅ and CAS number 460-73-1, is a hydrofluorocarbon (HFC) developed as a non-ozone-depleting substitute for chlorofluorocarbons (CFCs) and hydrochlorofluorocarbons (HCFCs) . It is widely used in foam blowing, refrigeration, solvent applications, and as a heat transfer medium . Its thermodynamic properties, including vapor pressures and phase behavior, have been extensively studied through fundamental equations of state . HFC-245fa exhibits low acute toxicity but has been associated with cardiotoxicity in rats due to minor metabolites like 3,3,3-trifluoropropanoic acid .
Preparation Methods
Liquid-Phase Fluorination of 1,1,1,3,3-Pentachloropropane
The reaction of 1,1,1,3,3-pentachloropropane (F240fa) with hydrogen fluoride (HF) in the liquid phase represents a foundational method for HFC-245fa synthesis. This approach, detailed in FR2768727A1, employs catalysts such as antimony pentachloride (SbCl₅) or chromium-based compounds under elevated pressures (1.0–1.5 MPa) and temperatures (60–140°C) . The process utilizes a dual-reaction-zone system:
-
Low-temperature zone (60–90°C): Initial fluorination occurs here, where F240fa reacts with HF to form intermediates like 3-chloro-1,1,1,3-tetrafluoropropane (F244).
-
High-temperature zone (90–140°C): Further fluorination converts F244 into HFC-245fa, achieving a molar HF-to-F240fa ratio of 6:1 to 15:1 .
This method achieves yields exceeding 85%, with unreacted HF recycled to minimize waste . However, challenges include catalyst deactivation due to chloride byproducts and the need for corrosion-resistant reactors (e.g., Inconel or Monel) .
Vapor-Phase Catalytic Fluorination
Vapor-phase fluorination, as outlined in EP0877009A1, bypasses liquid-phase limitations by operating at higher temperatures (150–250°C) and atmospheric pressure. The process occurs in two stages:
First-Stage Reaction
1,1,1,3,3-Pentachloropropane reacts with HF over a chromium oxide (Cr₂O₃) catalyst to produce 1,1,1-trifluoro-3-chloro-2-propene (CF₃CHClCH₂) as the primary intermediate . Excess HF (5–20 molar equivalents) ensures complete chlorination, while byproduct HCl is removed via scrubbing .
Second-Stage Reaction
The intermediate undergoes further fluorination at 200–300°C with a fluorinated alumina catalyst, yielding HFC-245fa. This step achieves >90% selectivity, with minor byproducts such as 1,1,1,3-tetrafluoropropene (CF₃CHFCH₂) .
Advantages:
-
Reduced catalyst fouling due to gaseous reactants.
-
Continuous production capabilities.
Multi-Step Processes via Intermediate Compounds
US5895825A discloses a two-step method starting from 1,1,1-trifluoro-3-chloro-2-propene (1233zd):
Step 1: Synthesis of 1,1,1,3-Tetrafluoro-2-propene (1234ze)
1233zd reacts with HF at 100–150°C in the presence of SbCl₅, yielding 1234ze. This exothermic reaction requires careful temperature control to prevent over-fluorination .
Step 2: Conversion to HFC-245fa
1234ze is hydrogenated using palladium-on-carbon (Pd/C) under 10–30 bar H₂ pressure, producing HFC-245fa with >95% purity. Distillation separates unreacted HF and intermediates .
Key Data:
Parameter | Step 1 | Step 2 |
---|---|---|
Temperature (°C) | 120–140 | 80–100 |
Pressure (bar) | 1–5 | 10–30 |
Catalyst | SbCl₅ | Pd/C |
Yield (%) | 78 | 92 |
Catalytic Systems and Optimization
Catalyst selection critically impacts reaction efficiency. Antimony-based catalysts (SbCl₅, SbF₅) dominate industrial processes due to their high activity, though chromium oxide (Cr₂O₃) and fluorinated alumina offer alternatives for vapor-phase systems . Catalyst lifespan varies:
Environmental and Economic Considerations
HFC-245fa production generates HCl and minor fluorinated byproducts (e.g., CF₃CHF₂). Modern plants integrate HCl recycling via oxychlorination processes, reducing waste . Economically, vapor-phase methods are preferred for lower operational costs, while liquid-phase systems suit small-scale production .
Chemical Reactions Analysis
Stability and Reactivity
Under normal storage and usage conditions, hazardous reactions are not expected to occur . 1,1,1,3,3-Pentafluoropropane is normally stable, even when exposed to fire, and does not react with water .
Atmospheric Degradation
The atmospheric degradation of this compound leads to the formation of carbon dioxide () and hydrogen fluoride () as the primary ultimate degradation products . Non-radical intermediates include carbonyl difluoride (), trifluoroacetaldehyde (fluoral, ), and trifluoromethanol () .
The degradation mechanism involves the formation of peroxynitrates (, , and ) and hydroperoxides (, , and ), which are short-lived intermediates that undergo photolysis, thermal decomposition, or reaction with hydroxyl radicals (), leading to the regeneration of peroxy radicals () or the formation of alkoxy radicals () .
Carbonyl difluoride () and trifluoromethanol () formed as intermediates are taken up by cloud droplets over days to weeks and hydrolyzed to and . Photolysis is a major pathway for , leading to the radical and ultimately to and .
Tropospheric Degradation Mechanism for HFC-245fa
Intermediate | Reaction |
---|---|
Peroxynitrates | , and → Photolysis, thermal decomposition or reaction with - OH, leading to regeneration of peroxy radicals or formation of alkoxy radicals. |
Hydroperoxides | , and → Photolysis, thermal decomposition or reaction with - OH, leading to regeneration of peroxy radicals or formation of alkoxy radicals. |
Carbonyl Difluoride | + → + 2 |
Trifluoromethanol | + → Hydrolyzed to and |
Trifluoroacetaldehyde | → Photolysis → → + |
Biotransformation
This compound is metabolized at low rates in vivo and in vitro . In rats, the major urinary metabolites identified are trifluoroacetic acid and inorganic fluoride. Minor metabolites include 3,3,3-trifluoropropanoic acid and this compound-2-ol . The extent of biotransformation after inhalation depends on the exposure concentrations .
In rat and human liver microsomes, this compound is biotransformed via a cytochrome P450-dependent reaction into trifluoroacetic acid and 3,3,3-trifluoropropanoic acid .
Metabolites of this compound
Human Exposure Studies
Toxicokinetic studies in humans have been conducted with inhaled this compound (HFC-245fa) . Metabolites such as fluoride, 3,3,3-trifluoropropionic acid (TFPA), and trifluoroacetic acid (TFAA) have been analyzed in urine samples following exposure .
Scientific Research Applications
Key Applications
-
Blowing Agent
- 1,1,1,3,3-Pentafluoropropane is primarily used as a blowing agent in the production of polyurethane foams. When heated, it transitions from liquid to gas, creating bubbles that expand and solidify into cellular structures. This property is crucial for manufacturing insulation panels and spray foam insulation that provide thermal insulation and sound absorption .
-
Refrigerant
- Due to its favorable thermodynamic properties and low boiling point, this compound serves as an effective refrigerant in air conditioning systems and refrigerators. It is considered a non-ozone-depleting alternative to traditional chlorofluorocarbons (CFCs), aligning with environmental regulations aimed at reducing ozone layer depletion .
- Aerosol Propellant
- Fire Suppression
- Heat Transfer Fluid
- Insulating Gas
Case Study 1: Use as a Blowing Agent
A study conducted on the efficiency of this compound as a blowing agent demonstrated its effectiveness in producing polyurethane foams with superior thermal insulation properties compared to other agents. The research highlighted the compound's ability to maintain structural integrity while minimizing environmental impact due to its low global warming potential.
Case Study 2: Refrigeration Applications
Research published in various journals has shown that replacing traditional refrigerants with this compound in refrigeration systems significantly reduces greenhouse gas emissions while maintaining energy efficiency. This transition aligns with international efforts to phase out harmful substances under the Montreal Protocol.
Mechanism of Action
The mechanism by which pentafluoropropane exerts its effects involves its interaction with various molecular targets and pathways. For example, in its use as a vapocoolant spray, it works by rapidly evaporating and absorbing heat from the skin, thereby numbing the area . The compound’s unique chemical structure allows it to interact with specific molecular targets, leading to its desired effects.
Comparison with Similar Compounds
Structural and Functional Analogues
HFC-245fa is compared to several related fluorinated compounds, including isomers, homologues, and substitutes:
Key Differences
- Reactivity : HFC-245fa exhibits lower reactivity in hydrodefluorination compared to its isomer HFC-245ca (1,1,1,2,3-pentafluoropropane). Silane (HSiEt₃) is required to activate the CHF₂ group in HFC-245fa, whereas HFC-245ca reacts spontaneously under similar conditions .
- HFC-245fa’s GWP (~1,030) is lower than R134a but higher than R365mfc .
- Applications : HFC-245fa and R365mfc dominate foam blowing, whereas R134a is preferred in refrigeration. HCFC-225cb, an older solvent, is being phased out due to its ODP .
Detailed Research Findings
Toxicity and Biotransformation
- Metabolism: In rats and humans, HFC-245fa undergoes cytochrome P450-dependent biotransformation to trifluoroacetic acid (TFA) and 3,3,3-trifluoropropanoic acid (TFPA). TFPA is linked to cardiotoxicity in rats at high exposure levels (≥50,000 ppm) .
- Human Disposition : Less than 1% of inhaled HFC-245fa is metabolized in humans, with rapid renal excretion of unmetabolized compound .
Thermodynamic Properties
HFC-245fa’s vapor pressures and phase equilibria are well-characterized, with critical temperatures and pressures modeled by Akasaka et al. (2015) . Its thermal conductivity and viscosity are critical for optimizing heat pump and refrigeration cycles .
Biological Activity
1,1,1,3,3-Pentafluoropropane (HFC-245fa) is a hydrofluorocarbon that has garnered attention as a potential substitute for ozone-depleting substances like chlorofluorocarbons (CFCs). Its biological activity, particularly its toxicological profile and metabolic pathways, is essential for understanding its safety and efficacy in various applications. This article synthesizes findings from diverse studies to provide a comprehensive overview of the biological activity of HFC-245fa.
HFC-245fa is primarily utilized as a foam-blowing agent and refrigerant. It is characterized by its low global warming potential and negligible ozone depletion potential. The compound's chemical structure contributes to its unique properties, making it suitable for various industrial applications.
In Vivo Studies
Research indicates that HFC-245fa is metabolized at low rates in both in vivo and in vitro settings. In a study involving rats exposed to high concentrations (up to 50,000 ppm) for extended periods (90 days), the primary toxic effect observed was an increased incidence of diffuse myocarditis. This condition was linked to the formation of toxic metabolites during biotransformation processes .
Key Findings:
- Metabolites Identified: Major urinary metabolites include trifluoroacetic acid (TFAA) and 3,3,3-trifluoropropanoic acid (TFPA), with TFAA being formed at significantly higher rates in rat liver microsomes compared to human microsomes .
- Biotransformation Mechanism: The metabolism of HFC-245fa occurs via cytochrome P450-dependent reactions, leading to the production of TFAA and TFPA. The rates of metabolite formation vary significantly between species .
Metabolite | Formation Rate in Rat Liver Microsomes | Formation Rate in Human Liver Microsomes |
---|---|---|
Trifluoroacetic Acid (TFAA) | 99.2 ± 20.5 pmol/(mg protein·min) | 0 - 11.6 pmol/(mg protein·min) |
3,3,3-Trifluoropropanoic Acid (TFPA) | 17.5 ± 4.0 pmol/(mg protein·min) | 0.7 - 7.6 pmol/(mg protein·min) |
Human Exposure Studies
A study conducted on healthy volunteers exposed to varying concentrations of HFC-245fa revealed insights into its toxicokinetics. Participants were exposed to concentrations of 0, 100, or 300 ppm for two hours while engaging in light exercise. Blood and urine samples were collected post-exposure to analyze levels of HFC-245fa and its metabolites .
Observations:
- Symptoms Reported: Participants reported symptoms such as irritation and central nervous system effects, which were assessed using visual analogue scales.
- Biochemical Analysis: Serum biochemistry showed no significant alterations in liver function tests post-exposure, indicating a low risk of acute toxicity at tested levels .
Case Studies
-
Cardiotoxicity in Rats:
- In a long-term exposure study on rats, histopathological examinations revealed myocarditis but no significant damage to other organs at lower exposure levels (10,000 ppm). This finding suggests that while HFC-245fa has a low overall toxicity profile, it may pose specific risks related to cardiac health under certain exposure conditions .
- Comparative Toxicity with Other Hydrofluorocarbons:
Q & A
Basic Research Questions
Q. What methodologies are recommended for optimizing gas-phase catalytic fluorination of 1,1,1,3,3-pentafluoropropane (HFC-245fa)?
To optimize gas-phase catalytic fluorination, use chromium-based catalysts (e.g., Cr₂O₃/AlF₃) at 250–350°C with a molar excess of hydrogen fluoride (HF) to 1,1,1,3,3-pentachloropropane (HCC-240fa) (≥5:1). Reaction efficiency improves with controlled residence time (10–30 seconds) and staged temperature gradients to minimize side products like 1-chloro-3,3,3-trifluoropropene (CF₃CH=CHCl). Post-reaction separation via fractional distillation or extractive distillation is critical for isolating HFC-245fa .
Q. How can researchers analyze and quantify impurities (e.g., halo-olefins) in HFC-245fa during synthesis?
Use gas chromatography-mass spectrometry (GC-MS) with a polar capillary column (e.g., DB-624) and electron capture detection (ECD) for high sensitivity to fluorinated impurities. Calibrate with standards of common by-products like trans-1-chloro-3,3,3-trifluoropropene. For quantification, employ internal standards (e.g., 1,2-dichloroethane) and validate recovery rates ≥95% .
Q. What experimental parameters influence the azeotropic behavior of HFC-245fa with trans-1,2-dichloroethylene?
Azeotropic mixtures (80–99.9 wt% HFC-245fa) exhibit constant boiling points (~15.1°C at 760 mmHg). Key parameters include vapor-liquid equilibrium (VLE) measurements at varying pressures and temperatures. Use a recirculating still to determine phase diagrams and validate with predictive models (e.g., UNIQUAC). This aids in designing separation protocols for solvent recovery .
Advanced Research Questions
Q. How can activated carbons be tailored to remove halo-olefinic impurities from HFC-245fa?
Activated carbons with high micropore volume (~0.5 cm³/g) and surface area (>1,200 m²/g) preferentially adsorb impurities like CF₃CH=CHCl. Modify carbons via acid washing (HCl/HNO₃) to enhance surface oxygen groups, improving adsorption capacity. Conduct breakthrough experiments in fixed-bed reactors at 25–40°C and 1–3 bar to optimize adsorption kinetics .
Q. What mechanistic insights explain the catalytic dehydrochlorination of HFC-245fa to 1,3,3,3-tetrafluoropropene (HFO-1234ze)?
The reaction proceeds via an E2 elimination mechanism, where HCl abstraction is facilitated by Lewis acid catalysts (e.g., AlCl₃ or Cr₂O₃). Density functional theory (DFT) studies suggest transition states with partial C–Cl bond cleavage and simultaneous H⁺ transfer. Optimize selectivity (>90%) by controlling HCl partial pressure (10–20 mol%) and catalyst bed geometry to minimize coking .
Q. How can researchers resolve contradictions in reported reaction yields for HFC-245fa synthesis via liquid-phase fluorination?
Discrepancies arise from varying HF stoichiometry (3–10 mol equivalents) and catalyst stability (e.g., SbF₅ vs. SnCl₄). Replicate studies under identical conditions (T = 80–120°C, P = 5–10 bar) and characterize catalyst deactivation via X-ray diffraction (XRD) and thermogravimetric analysis (TGA). Cross-validate yield data using in-line IR spectroscopy to monitor intermediate formation .
Q. What methodologies assess the environmental impact of HFC-245fa leakage in lifecycle analyses (LCAs)?
Use the Tool for Reduction and Assessment of Chemicals and Other Environmental Impacts (TRACI) to calculate global warming potential (GWP) based on radiative forcing (1,030 CO₂-eq over 100 years). Incorporate atmospheric lifetime (7.6 years) and degradation products (trifluoroacetic acid) from OH· radical reaction studies. Validate with chamber experiments measuring hydrolysis rates under UV exposure .
Properties
IUPAC Name |
1,1,1,3,3-pentafluoropropane | |
---|---|---|
Source | PubChem | |
URL | https://pubchem.ncbi.nlm.nih.gov | |
Description | Data deposited in or computed by PubChem | |
InChI |
InChI=1S/C3H3F5/c4-2(5)1-3(6,7)8/h2H,1H2 | |
Source | PubChem | |
URL | https://pubchem.ncbi.nlm.nih.gov | |
Description | Data deposited in or computed by PubChem | |
InChI Key |
MSSNHSVIGIHOJA-UHFFFAOYSA-N | |
Source | PubChem | |
URL | https://pubchem.ncbi.nlm.nih.gov | |
Description | Data deposited in or computed by PubChem | |
Canonical SMILES |
C(C(F)F)C(F)(F)F | |
Source | PubChem | |
URL | https://pubchem.ncbi.nlm.nih.gov | |
Description | Data deposited in or computed by PubChem | |
Molecular Formula |
C3H3F5 | |
Source | PubChem | |
URL | https://pubchem.ncbi.nlm.nih.gov | |
Description | Data deposited in or computed by PubChem | |
DSSTOX Substance ID |
DTXSID6052110 | |
Record name | 1,1,1,3,3-Pentafluoropropane | |
Source | EPA DSSTox | |
URL | https://comptox.epa.gov/dashboard/DTXSID6052110 | |
Description | DSSTox provides a high quality public chemistry resource for supporting improved predictive toxicology. | |
Molecular Weight |
134.05 g/mol | |
Source | PubChem | |
URL | https://pubchem.ncbi.nlm.nih.gov | |
Description | Data deposited in or computed by PubChem | |
Physical Description |
Gas or Vapor; Liquid, Colorless compressed gas or liquefied gas with a slight ethereal odor; [CHEMINFO] Ether-like odor; [MSDSonline] | |
Record name | Propane, 1,1,1,3,3-pentafluoro- | |
Source | EPA Chemicals under the TSCA | |
URL | https://www.epa.gov/chemicals-under-tsca | |
Description | EPA Chemicals under the Toxic Substances Control Act (TSCA) collection contains information on chemicals and their regulations under TSCA, including non-confidential content from the TSCA Chemical Substance Inventory and Chemical Data Reporting. | |
Record name | 1,1,1,3,3-Pentafluoropropane | |
Source | Haz-Map, Information on Hazardous Chemicals and Occupational Diseases | |
URL | https://haz-map.com/Agents/7874 | |
Description | Haz-Map® is an occupational health database designed for health and safety professionals and for consumers seeking information about the adverse effects of workplace exposures to chemical and biological agents. | |
Explanation | Copyright (c) 2022 Haz-Map(R). All rights reserved. Unless otherwise indicated, all materials from Haz-Map are copyrighted by Haz-Map(R). No part of these materials, either text or image may be used for any purpose other than for personal use. Therefore, reproduction, modification, storage in a retrieval system or retransmission, in any form or by any means, electronic, mechanical or otherwise, for reasons other than personal use, is strictly prohibited without prior written permission. | |
CAS No. |
460-73-1 | |
Record name | 1,1,1,3,3-Pentafluoropropane | |
Source | CAS Common Chemistry | |
URL | https://commonchemistry.cas.org/detail?cas_rn=460-73-1 | |
Description | CAS Common Chemistry is an open community resource for accessing chemical information. Nearly 500,000 chemical substances from CAS REGISTRY cover areas of community interest, including common and frequently regulated chemicals, and those relevant to high school and undergraduate chemistry classes. This chemical information, curated by our expert scientists, is provided in alignment with our mission as a division of the American Chemical Society. | |
Explanation | The data from CAS Common Chemistry is provided under a CC-BY-NC 4.0 license, unless otherwise stated. | |
Record name | HFC 245fa | |
Source | ChemIDplus | |
URL | https://pubchem.ncbi.nlm.nih.gov/substance/?source=chemidplus&sourceid=0000460731 | |
Description | ChemIDplus is a free, web search system that provides access to the structure and nomenclature authority files used for the identification of chemical substances cited in National Library of Medicine (NLM) databases, including the TOXNET system. | |
Record name | Pentafluoropropane | |
Source | DrugBank | |
URL | https://www.drugbank.ca/drugs/DB13117 | |
Description | The DrugBank database is a unique bioinformatics and cheminformatics resource that combines detailed drug (i.e. chemical, pharmacological and pharmaceutical) data with comprehensive drug target (i.e. sequence, structure, and pathway) information. | |
Explanation | Creative Common's Attribution-NonCommercial 4.0 International License (http://creativecommons.org/licenses/by-nc/4.0/legalcode) | |
Record name | Propane, 1,1,1,3,3-pentafluoro- | |
Source | EPA Chemicals under the TSCA | |
URL | https://www.epa.gov/chemicals-under-tsca | |
Description | EPA Chemicals under the Toxic Substances Control Act (TSCA) collection contains information on chemicals and their regulations under TSCA, including non-confidential content from the TSCA Chemical Substance Inventory and Chemical Data Reporting. | |
Record name | 1,1,1,3,3-Pentafluoropropane | |
Source | EPA DSSTox | |
URL | https://comptox.epa.gov/dashboard/DTXSID6052110 | |
Description | DSSTox provides a high quality public chemistry resource for supporting improved predictive toxicology. | |
Record name | Propane, 1,1,1,3,3-pentafluoro | |
Source | European Chemicals Agency (ECHA) | |
URL | https://echa.europa.eu/substance-information/-/substanceinfo/100.114.041 | |
Description | The European Chemicals Agency (ECHA) is an agency of the European Union which is the driving force among regulatory authorities in implementing the EU's groundbreaking chemicals legislation for the benefit of human health and the environment as well as for innovation and competitiveness. | |
Explanation | Use of the information, documents and data from the ECHA website is subject to the terms and conditions of this Legal Notice, and subject to other binding limitations provided for under applicable law, the information, documents and data made available on the ECHA website may be reproduced, distributed and/or used, totally or in part, for non-commercial purposes provided that ECHA is acknowledged as the source: "Source: European Chemicals Agency, http://echa.europa.eu/". Such acknowledgement must be included in each copy of the material. ECHA permits and encourages organisations and individuals to create links to the ECHA website under the following cumulative conditions: Links can only be made to webpages that provide a link to the Legal Notice page. | |
Record name | PENTAFLUOROPROPANE | |
Source | FDA Global Substance Registration System (GSRS) | |
URL | https://gsrs.ncats.nih.gov/ginas/app/beta/substances/TA9UOF49CY | |
Description | The FDA Global Substance Registration System (GSRS) enables the efficient and accurate exchange of information on what substances are in regulated products. Instead of relying on names, which vary across regulatory domains, countries, and regions, the GSRS knowledge base makes it possible for substances to be defined by standardized, scientific descriptions. | |
Explanation | Unless otherwise noted, the contents of the FDA website (www.fda.gov), both text and graphics, are not copyrighted. They are in the public domain and may be republished, reprinted and otherwise used freely by anyone without the need to obtain permission from FDA. Credit to the U.S. Food and Drug Administration as the source is appreciated but not required. | |
Retrosynthesis Analysis
AI-Powered Synthesis Planning: Our tool employs the Template_relevance Pistachio, Template_relevance Bkms_metabolic, Template_relevance Pistachio_ringbreaker, Template_relevance Reaxys, Template_relevance Reaxys_biocatalysis model, leveraging a vast database of chemical reactions to predict feasible synthetic routes.
One-Step Synthesis Focus: Specifically designed for one-step synthesis, it provides concise and direct routes for your target compounds, streamlining the synthesis process.
Accurate Predictions: Utilizing the extensive PISTACHIO, BKMS_METABOLIC, PISTACHIO_RINGBREAKER, REAXYS, REAXYS_BIOCATALYSIS database, our tool offers high-accuracy predictions, reflecting the latest in chemical research and data.
Strategy Settings
Precursor scoring | Relevance Heuristic |
---|---|
Min. plausibility | 0.01 |
Model | Template_relevance |
Template Set | Pistachio/Bkms_metabolic/Pistachio_ringbreaker/Reaxys/Reaxys_biocatalysis |
Top-N result to add to graph | 6 |
Feasible Synthetic Routes
Disclaimer and Information on In-Vitro Research Products
Please be aware that all articles and product information presented on BenchChem are intended solely for informational purposes. The products available for purchase on BenchChem are specifically designed for in-vitro studies, which are conducted outside of living organisms. In-vitro studies, derived from the Latin term "in glass," involve experiments performed in controlled laboratory settings using cells or tissues. It is important to note that these products are not categorized as medicines or drugs, and they have not received approval from the FDA for the prevention, treatment, or cure of any medical condition, ailment, or disease. We must emphasize that any form of bodily introduction of these products into humans or animals is strictly prohibited by law. It is essential to adhere to these guidelines to ensure compliance with legal and ethical standards in research and experimentation.