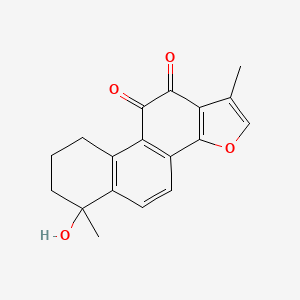
Tanshinol B
Overview
Description
. This compound is a flavonoid with an aromatic glycoside structure and appears as a white crystalline powder. It is soluble in ethanol and dimethylformamide and is relatively stable under neutral and mildly alkaline conditions but may decompose in strongly acidic environments . Tanshinol B exhibits various biological activities, including anti-inflammatory, antioxidant, and antifibrinolytic properties .
Mechanism of Action
Target of Action
Tanshinol B, also known as Przewaquinone C, is a bioactive compound isolated from the roots of Salvia miltiorrhiza . It has been found to interact with several key proteins such as AKT1, IL-6, and EGFR . These proteins play crucial roles in various biological processes, including immune regulation, cancer progression, and lipid metabolism .
Mode of Action
This compound exerts its effects through multiple mechanisms. It has been reported to induce apoptosis in Colo 205 cells through both mitochondrial-mediated intrinsic cell-death pathways and p21-mediated G0/G1 cell cycle arrest . Furthermore, it has been found to regulate key proteins such as AKT1, IL-6, and EGFR , which are involved in various biological processes.
Biochemical Pathways
The biochemical pathways affected by this compound are diverse. It has been found to be involved in pathways such as immune regulation, cancer and lipid metabolism, lipid and atherosclerosis, chemical carcinogenesis-receptor activation pathways, and IL-17 signaling pathway . These pathways are crucial for various physiological processes and disease states.
Pharmacokinetics
The pharmacokinetics of this compound have been studied in rat plasma after oral administration . The population pharmacokinetic models of this compound were best described by a one-compartment model with linear elimination and first-order absorption . These properties impact the bioavailability of this compound, influencing its therapeutic effects.
Result of Action
This compound has been found to have various molecular and cellular effects. It has been shown to downregulate the contents of interleukin 6, tumor necrosis factor alpha, and prostaglandin E2 in both saliva and blood, decrease the infiltration of inflammatory cells, and restore the destruction of alveolar bone . These effects highlight the therapeutic value of this compound in conditions such as periodontitis .
Preparation Methods
Tanshinol B can be prepared through both natural extraction and synthetic routes. The natural extraction involves grinding, soaking, and extracting the raw Danshen material, followed by solvent extraction and crystallization to obtain this compound .
In terms of synthetic routes, a concise and efficient approach has been established for the total synthesis of this compound. This method involves an ultrasound-promoted cycloaddition as a key step, achieving a 50% overall yield over three steps . This synthetic route is more step-economic compared to previously reported strategies, significantly improving synthetic efficiency .
Chemical Reactions Analysis
Tanshinol B undergoes various chemical reactions, including oxidation, reduction, and substitution. Common reagents and conditions used in these reactions include:
Oxidation: this compound can be oxidized using reagents such as potassium permanganate or hydrogen peroxide under acidic conditions.
Reduction: Reduction reactions can be carried out using reagents like sodium borohydride or lithium aluminum hydride.
Substitution: Substitution reactions often involve nucleophiles such as halides or amines under basic conditions.
The major products formed from these reactions depend on the specific reagents and conditions used. For example, oxidation may yield quinones, while reduction can produce alcohols or hydrocarbons .
Scientific Research Applications
Tanshinol B has a wide range of scientific research applications across various fields:
Chemistry: It is used as a reference compound in analytical chemistry for the identification and quantification of similar flavonoids.
Comparison with Similar Compounds
. Similar compounds include:
Tanshinone I: Known for its cytotoxic activity and ability to induce apoptosis.
Tanshinone IIA: Exhibits strong anti-inflammatory and antioxidant properties.
Cryptotanshinone: Noted for its antibacterial and antineoplastic activities.
Compared to these similar compounds, Tanshinol B is unique due to its specific glycoside structure and its stability under neutral and mildly alkaline conditions . This structural uniqueness contributes to its distinct biological activities and potential therapeutic applications.
Properties
IUPAC Name |
6-hydroxy-1,6-dimethyl-8,9-dihydro-7H-naphtho[1,2-g][1]benzofuran-10,11-dione | |
---|---|---|
Source | PubChem | |
URL | https://pubchem.ncbi.nlm.nih.gov | |
Description | Data deposited in or computed by PubChem | |
InChI |
InChI=1S/C18H16O4/c1-9-8-22-17-11-5-6-12-10(4-3-7-18(12,2)21)14(11)16(20)15(19)13(9)17/h5-6,8,21H,3-4,7H2,1-2H3 | |
Source | PubChem | |
URL | https://pubchem.ncbi.nlm.nih.gov | |
Description | Data deposited in or computed by PubChem | |
InChI Key |
JVRKHBLVECIWMZ-UHFFFAOYSA-N | |
Source | PubChem | |
URL | https://pubchem.ncbi.nlm.nih.gov | |
Description | Data deposited in or computed by PubChem | |
Canonical SMILES |
CC1=COC2=C1C(=O)C(=O)C3=C2C=CC4=C3CCCC4(C)O | |
Source | PubChem | |
URL | https://pubchem.ncbi.nlm.nih.gov | |
Description | Data deposited in or computed by PubChem | |
Molecular Formula |
C18H16O4 | |
Source | PubChem | |
URL | https://pubchem.ncbi.nlm.nih.gov | |
Description | Data deposited in or computed by PubChem | |
DSSTOX Substance ID |
DTXSID90914288 | |
Record name | 6-Hydroxy-1,6-dimethyl-6,7,8,9-tetrahydrophenanthro[1,2-b]furan-10,11-dione | |
Source | EPA DSSTox | |
URL | https://comptox.epa.gov/dashboard/DTXSID90914288 | |
Description | DSSTox provides a high quality public chemistry resource for supporting improved predictive toxicology. | |
Molecular Weight |
296.3 g/mol | |
Source | PubChem | |
URL | https://pubchem.ncbi.nlm.nih.gov | |
Description | Data deposited in or computed by PubChem | |
CAS No. |
96839-29-1 | |
Record name | Przewaquinone C | |
Source | ChemIDplus | |
URL | https://pubchem.ncbi.nlm.nih.gov/substance/?source=chemidplus&sourceid=0096839291 | |
Description | ChemIDplus is a free, web search system that provides access to the structure and nomenclature authority files used for the identification of chemical substances cited in National Library of Medicine (NLM) databases, including the TOXNET system. | |
Record name | 6-Hydroxy-1,6-dimethyl-6,7,8,9-tetrahydrophenanthro[1,2-b]furan-10,11-dione | |
Source | EPA DSSTox | |
URL | https://comptox.epa.gov/dashboard/DTXSID90914288 | |
Description | DSSTox provides a high quality public chemistry resource for supporting improved predictive toxicology. | |
Retrosynthesis Analysis
AI-Powered Synthesis Planning: Our tool employs the Template_relevance Pistachio, Template_relevance Bkms_metabolic, Template_relevance Pistachio_ringbreaker, Template_relevance Reaxys, Template_relevance Reaxys_biocatalysis model, leveraging a vast database of chemical reactions to predict feasible synthetic routes.
One-Step Synthesis Focus: Specifically designed for one-step synthesis, it provides concise and direct routes for your target compounds, streamlining the synthesis process.
Accurate Predictions: Utilizing the extensive PISTACHIO, BKMS_METABOLIC, PISTACHIO_RINGBREAKER, REAXYS, REAXYS_BIOCATALYSIS database, our tool offers high-accuracy predictions, reflecting the latest in chemical research and data.
Strategy Settings
Precursor scoring | Relevance Heuristic |
---|---|
Min. plausibility | 0.01 |
Model | Template_relevance |
Template Set | Pistachio/Bkms_metabolic/Pistachio_ringbreaker/Reaxys/Reaxys_biocatalysis |
Top-N result to add to graph | 6 |
Feasible Synthetic Routes
Disclaimer and Information on In-Vitro Research Products
Please be aware that all articles and product information presented on BenchChem are intended solely for informational purposes. The products available for purchase on BenchChem are specifically designed for in-vitro studies, which are conducted outside of living organisms. In-vitro studies, derived from the Latin term "in glass," involve experiments performed in controlled laboratory settings using cells or tissues. It is important to note that these products are not categorized as medicines or drugs, and they have not received approval from the FDA for the prevention, treatment, or cure of any medical condition, ailment, or disease. We must emphasize that any form of bodily introduction of these products into humans or animals is strictly prohibited by law. It is essential to adhere to these guidelines to ensure compliance with legal and ethical standards in research and experimentation.