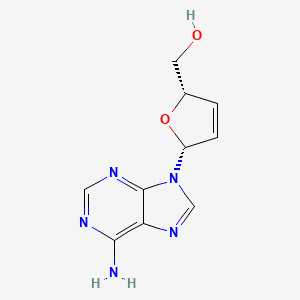
2',3'-Dideoxy-2',3'-didehydroadenosine
Overview
Description
Scientific Research Applications
Beta-L-D4A has a wide range of scientific research applications, including:
Chemistry: Used as a model compound for studying nucleoside analogs and their chemical properties.
Biology: Investigated for its effects on DNA polymerase and other enzymes involved in DNA replication.
Medicine: Studied for its antiviral properties, particularly against hepatitis B virus and human immunodeficiency virus
Industry: Used in the development of antiviral drugs and other therapeutic agents.
Mechanism of Action
Beta-L-D4A exerts its effects by inhibiting viral DNA polymerase, an enzyme crucial for viral replication. The compound acts as a chain terminator, preventing the elongation of viral DNA and thereby inhibiting viral replication. This mechanism is particularly effective against hepatitis B virus and human immunodeficiency virus .
Biochemical Analysis
Biochemical Properties
2’,3’-Dideoxy-2’,3’-didehydroadenosine plays a crucial role in biochemical reactions, particularly in the inhibition of viral DNA synthesis. It interacts with viral DNA polymerase, an enzyme responsible for the replication of viral DNA. By binding to the DNA polymerase, 2’,3’-Dideoxy-2’,3’-didehydroadenosine blocks the incorporation of deoxycytidine into the viral DNA, thereby halting viral replication . This compound is active against both wild-type and mutant strains of hepatitis B virus . Notably, 2’,3’-Dideoxy-2’,3’-didehydroadenosine does not affect the synthesis of cellular DNA or RNA, nor does it inhibit cellular protein synthesis .
Cellular Effects
The effects of 2’,3’-Dideoxy-2’,3’-didehydroadenosine on various cell types and cellular processes are profound. This compound influences cell function by inhibiting viral replication, which in turn affects cell signaling pathways, gene expression, and cellular metabolism. In infected cells, 2’,3’-Dideoxy-2’,3’-didehydroadenosine disrupts the normal replication cycle of the virus, leading to a decrease in viral load and an improvement in cellular health . Additionally, this compound has been shown to have minimal cytotoxic effects on uninfected cells, making it a promising candidate for antiviral therapy .
Molecular Mechanism
At the molecular level, 2’,3’-Dideoxy-2’,3’-didehydroadenosine exerts its effects through several mechanisms. The primary mechanism involves the inhibition of viral DNA polymerase by binding to its active site . This binding prevents the incorporation of deoxycytidine into the growing viral DNA chain, effectively terminating DNA synthesis . Furthermore, 2’,3’-Dideoxy-2’,3’-didehydroadenosine may also induce conformational changes in the DNA polymerase, reducing its activity and stability . These interactions highlight the compound’s specificity and potency as an antiviral agent.
Temporal Effects in Laboratory Settings
In laboratory settings, the effects of 2’,3’-Dideoxy-2’,3’-didehydroadenosine have been observed to change over time. The compound is relatively stable under standard storage conditions (10°C - 25°C) and does not degrade rapidly . Long-term studies have shown that 2’,3’-Dideoxy-2’,3’-didehydroadenosine maintains its antiviral activity over extended periods, with no significant loss of potency .
Dosage Effects in Animal Models
The effects of 2’,3’-Dideoxy-2’,3’-didehydroadenosine vary with different dosages in animal models. At low to moderate doses, the compound effectively inhibits viral replication without causing significant toxicity . At high doses, 2’,3’-Dideoxy-2’,3’-didehydroadenosine may exhibit toxic effects, including hepatotoxicity and nephrotoxicity . These adverse effects highlight the importance of determining the optimal dosage for therapeutic use to maximize efficacy while minimizing toxicity.
Metabolic Pathways
2’,3’-Dideoxy-2’,3’-didehydroadenosine is involved in several metabolic pathways, primarily related to its antiviral activity. The compound is metabolized by cellular enzymes, including kinases that phosphorylate it to its active triphosphate form . This active form is then incorporated into viral DNA by the DNA polymerase, leading to chain termination . The metabolism of 2’,3’-Dideoxy-2’,3’-didehydroadenosine does not significantly affect cellular metabolic flux or metabolite levels, indicating its specificity for viral targets .
Transport and Distribution
Within cells and tissues, 2’,3’-Dideoxy-2’,3’-didehydroadenosine is transported and distributed through various mechanisms. The compound is taken up by cells via nucleoside transporters, which facilitate its entry into the cytoplasm . Once inside the cell, 2’,3’-Dideoxy-2’,3’-didehydroadenosine is distributed to different cellular compartments, where it exerts its antiviral effects . The localization and accumulation of the compound are influenced by its interactions with cellular binding proteins and transporters .
Subcellular Localization
The subcellular localization of 2’,3’-Dideoxy-2’,3’-didehydroadenosine is critical for its activity and function. The compound is primarily localized in the cytoplasm, where it interacts with viral DNA polymerase . Post-translational modifications and targeting signals may direct 2’,3’-Dideoxy-2’,3’-didehydroadenosine to specific compartments or organelles, enhancing its antiviral efficacy . Understanding the subcellular distribution of this compound can provide insights into its mechanism of action and potential therapeutic applications.
Preparation Methods
Synthetic Routes and Reaction Conditions: The synthesis of beta-L-D4A involves the dehydrogenation and deoxygenation of adenosine. The process typically includes the following steps:
Dehydrogenation: Adenosine is treated with a dehydrogenating agent to remove hydrogen atoms from the 2’ and 3’ positions.
Industrial Production Methods: Industrial production of beta-L-D4A follows similar synthetic routes but on a larger scale. The process involves:
Bulk Synthesis: Large quantities of adenosine are subjected to dehydrogenation and deoxygenation reactions under controlled conditions.
Purification: The crude product is purified using techniques such as ion exchange chromatography to obtain high-purity beta-L-D4A.
Chemical Reactions Analysis
Types of Reactions: Beta-L-D4A undergoes various chemical reactions, including:
Oxidation: Beta-L-D4A can be oxidized to form corresponding oxides.
Reduction: It can be reduced to form reduced derivatives.
Substitution: Beta-L-D4A can undergo substitution reactions where functional groups are replaced by other groups.
Common Reagents and Conditions:
Oxidation: Common oxidizing agents include potassium permanganate and hydrogen peroxide.
Reduction: Reducing agents such as sodium borohydride and lithium aluminum hydride are used.
Substitution: Substitution reactions often involve reagents like halogens and alkylating agents.
Major Products: The major products formed from these reactions include various derivatives of beta-L-D4A, which can have different biological activities and properties .
Comparison with Similar Compounds
Similar Compounds:
Lamivudine (3TC): Another nucleoside analog used in the treatment of hepatitis B virus and human immunodeficiency virus.
Adefovir: A nucleotide analog used for the treatment of hepatitis B virus.
Tenofovir: A nucleotide analog used for the treatment of hepatitis B virus and human immunodeficiency virus.
Comparison: Beta-L-D4A is unique in its structure and mechanism of action. It has shown higher potency and lower toxicity compared to lamivudine and other similar compounds. Its ability to inhibit viral replication at lower concentrations makes it a promising candidate for antiviral therapy .
Properties
IUPAC Name |
[5-(6-aminopurin-9-yl)-2,5-dihydrofuran-2-yl]methanol | |
---|---|---|
Details | Computed by Lexichem TK 2.7.0 (PubChem release 2021.05.07) | |
Source | PubChem | |
URL | https://pubchem.ncbi.nlm.nih.gov | |
Description | Data deposited in or computed by PubChem | |
InChI |
InChI=1S/C10H11N5O2/c11-9-8-10(13-4-12-9)15(5-14-8)7-2-1-6(3-16)17-7/h1-2,4-7,16H,3H2,(H2,11,12,13) | |
Details | Computed by InChI 1.0.6 (PubChem release 2021.05.07) | |
Source | PubChem | |
URL | https://pubchem.ncbi.nlm.nih.gov | |
Description | Data deposited in or computed by PubChem | |
InChI Key |
JFUOUIPRAAGUGF-UHFFFAOYSA-N | |
Details | Computed by InChI 1.0.6 (PubChem release 2021.05.07) | |
Source | PubChem | |
URL | https://pubchem.ncbi.nlm.nih.gov | |
Description | Data deposited in or computed by PubChem | |
Canonical SMILES |
C1=CC(OC1CO)N2C=NC3=C(N=CN=C32)N | |
Details | Computed by OEChem 2.3.0 (PubChem release 2021.05.07) | |
Source | PubChem | |
URL | https://pubchem.ncbi.nlm.nih.gov | |
Description | Data deposited in or computed by PubChem | |
Molecular Formula |
C10H11N5O2 | |
Details | Computed by PubChem 2.1 (PubChem release 2021.05.07) | |
Source | PubChem | |
URL | https://pubchem.ncbi.nlm.nih.gov | |
Description | Data deposited in or computed by PubChem | |
Molecular Weight |
233.23 g/mol | |
Details | Computed by PubChem 2.1 (PubChem release 2021.05.07) | |
Source | PubChem | |
URL | https://pubchem.ncbi.nlm.nih.gov | |
Description | Data deposited in or computed by PubChem | |
CAS No. |
7057-48-9 | |
Record name | 2',3'-Dideoxyadenosine | |
Source | DTP/NCI | |
URL | https://dtp.cancer.gov/dtpstandard/servlet/dwindex?searchtype=NSC&outputformat=html&searchlist=108602 | |
Description | The NCI Development Therapeutics Program (DTP) provides services and resources to the academic and private-sector research communities worldwide to facilitate the discovery and development of new cancer therapeutic agents. | |
Explanation | Unless otherwise indicated, all text within NCI products is free of copyright and may be reused without our permission. Credit the National Cancer Institute as the source. | |
Retrosynthesis Analysis
AI-Powered Synthesis Planning: Our tool employs the Template_relevance Pistachio, Template_relevance Bkms_metabolic, Template_relevance Pistachio_ringbreaker, Template_relevance Reaxys, Template_relevance Reaxys_biocatalysis model, leveraging a vast database of chemical reactions to predict feasible synthetic routes.
One-Step Synthesis Focus: Specifically designed for one-step synthesis, it provides concise and direct routes for your target compounds, streamlining the synthesis process.
Accurate Predictions: Utilizing the extensive PISTACHIO, BKMS_METABOLIC, PISTACHIO_RINGBREAKER, REAXYS, REAXYS_BIOCATALYSIS database, our tool offers high-accuracy predictions, reflecting the latest in chemical research and data.
Strategy Settings
Precursor scoring | Relevance Heuristic |
---|---|
Min. plausibility | 0.01 |
Model | Template_relevance |
Template Set | Pistachio/Bkms_metabolic/Pistachio_ringbreaker/Reaxys/Reaxys_biocatalysis |
Top-N result to add to graph | 6 |
Feasible Synthetic Routes
Disclaimer and Information on In-Vitro Research Products
Please be aware that all articles and product information presented on BenchChem are intended solely for informational purposes. The products available for purchase on BenchChem are specifically designed for in-vitro studies, which are conducted outside of living organisms. In-vitro studies, derived from the Latin term "in glass," involve experiments performed in controlled laboratory settings using cells or tissues. It is important to note that these products are not categorized as medicines or drugs, and they have not received approval from the FDA for the prevention, treatment, or cure of any medical condition, ailment, or disease. We must emphasize that any form of bodily introduction of these products into humans or animals is strictly prohibited by law. It is essential to adhere to these guidelines to ensure compliance with legal and ethical standards in research and experimentation.