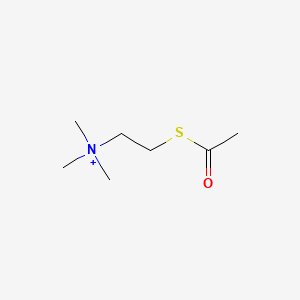
Acetylthiocholine
Overview
Description
Acetylthiocholine is an analog of acetylcholine, a neurotransmitter used at neuromuscular junctions to activate muscles. It is primarily used in scientific research, particularly in studies involving cholinesterase activity .
Preparation Methods
Acetylthiocholine can be synthesized through various methods. One common approach involves the reaction of thiocholine with acetic anhydride under controlled conditions. The reaction typically requires a catalyst and is carried out in an inert atmosphere to prevent oxidation . Industrial production methods often involve similar synthetic routes but on a larger scale, with additional purification steps to ensure the compound’s purity .
Chemical Reactions Analysis
Acetylthiocholine undergoes several types of chemical reactions:
Hydrolysis: This compound is hydrolyzed by acetylcholinesterase and butyrylcholinesterase to produce thiocholine and acetic acid.
Oxidation: Thiocholine, a product of this compound hydrolysis, can undergo oxidation to form disulfides.
Substitution: This compound can participate in substitution reactions, particularly in the presence of nucleophiles.
Common reagents used in these reactions include acetylcholinesterase, butyrylcholinesterase, and various nucleophiles. The major products formed from these reactions are thiocholine and acetic acid .
Scientific Research Applications
Acetylthiocholine is widely used in scientific research due to its role as a substrate for cholinesterase enzymes. Some key applications include:
Mechanism of Action
Acetylthiocholine exerts its effects primarily through its interaction with cholinesterase enzymes. When hydrolyzed by acetylcholinesterase or butyrylcholinesterase, it produces thiocholine and acetic acid. This hydrolysis reaction is crucial for terminating neurotransmission at cholinergic synapses . The molecular targets involved include the active sites of acetylcholinesterase and butyrylcholinesterase .
Comparison with Similar Compounds
Acetylthiocholine is similar to acetylcholine, but with a thioester linkage instead of an ester linkage. This modification makes it a useful analog for studying cholinesterase activity. Other similar compounds include:
Acetylcholine: The natural neurotransmitter with an ester linkage.
Butyrylcholine: Another cholinesterase substrate with a butyryl group instead of an acetyl group.
Thiocholine: A product of this compound hydrolysis, used in various biochemical assays.
This compound’s uniqueness lies in its thioester linkage, which allows for specific interactions with cholinesterase enzymes and makes it a valuable tool in scientific research .
Biological Activity
Acetylthiocholine (ATCh) is a synthetic analogue of acetylcholine, primarily used as a substrate in the study of cholinesterase enzymes. Its biological activity is crucial for understanding neurotransmission and enzyme kinetics, particularly in relation to acetylcholinesterase (AChE). This article explores the biological activity of this compound, including its interaction with AChE, kinetic properties, and implications for pharmacology and toxicology.
Overview of this compound
This compound is commonly used in biochemical assays to measure cholinesterase activity. It serves as a substrate for AChE, which catalyzes its hydrolysis into acetate and thiocholine. The reaction can be monitored spectrophotometrically, providing insights into enzyme kinetics and inhibition.
- Enzyme Interaction : this compound binds to two distinct sites on AChE: the catalytic site and the peripheral site. Studies indicate that mutations in the enzyme can significantly alter the binding affinity for ATCh, impacting the enzyme's turnover rate and overall activity .
- Kinetic Properties : The hydrolysis of this compound by AChE has been characterized using various methods, including calorimetry and spectrophotometry. The kinetic parameters such as (Michaelis constant) and (maximum velocity) are essential for understanding the efficiency of AChE with ATCh compared to natural substrates like acetylcholine .
Kinetic Parameters
The following table summarizes key kinetic parameters observed in studies involving this compound:
Study | Substrate | (mM) | (μmol/min/mg protein) | Inhibitor Used |
---|---|---|---|---|
This compound | 0.5 | 0.78 ± 0.28 | Carbaryl | |
Acetylcholine | 0.2 | 1.00 ± 0.30 | None |
Case Studies
- Inhibition Studies : Research has demonstrated that various pesticides inhibit cholinesterase activity when this compound is used as a substrate. For example, exposure to chlorpyrifos resulted in a significant decrease in AChE activity, indicating potential neurotoxic effects .
- Molecular Docking : In silico studies have shown that this compound interacts with the peripheral anionic site (PAS) of AChE, which is crucial for designing new inhibitors targeting Alzheimer's disease . These findings suggest that modifications to ATCh could enhance its efficacy as a therapeutic agent.
Implications for Pharmacology and Toxicology
This compound's role as a substrate in cholinesterase assays makes it invaluable in pharmacological research, particularly for developing drugs that target cholinergic pathways. Its use in toxicity studies highlights its importance in assessing the impact of environmental chemicals on enzyme function.
Properties
CAS No. |
4468-05-7 |
---|---|
Molecular Formula |
C7H16NOS+ |
Molecular Weight |
162.28 g/mol |
IUPAC Name |
2-acetylsulfanylethyl(trimethyl)azanium |
InChI |
InChI=1S/C7H16NOS/c1-7(9)10-6-5-8(2,3)4/h5-6H2,1-4H3/q+1 |
InChI Key |
GFFIJCYHQYHUHB-UHFFFAOYSA-N |
SMILES |
CC(=O)SCC[N+](C)(C)C |
Canonical SMILES |
CC(=O)SCC[N+](C)(C)C |
Key on ui other cas no. |
4468-05-7 |
Synonyms |
(2-Mercaptoethyl)trimethylammonium Acetate Acetylthiocholine |
Origin of Product |
United States |
Retrosynthesis Analysis
AI-Powered Synthesis Planning: Our tool employs the Template_relevance Pistachio, Template_relevance Bkms_metabolic, Template_relevance Pistachio_ringbreaker, Template_relevance Reaxys, Template_relevance Reaxys_biocatalysis model, leveraging a vast database of chemical reactions to predict feasible synthetic routes.
One-Step Synthesis Focus: Specifically designed for one-step synthesis, it provides concise and direct routes for your target compounds, streamlining the synthesis process.
Accurate Predictions: Utilizing the extensive PISTACHIO, BKMS_METABOLIC, PISTACHIO_RINGBREAKER, REAXYS, REAXYS_BIOCATALYSIS database, our tool offers high-accuracy predictions, reflecting the latest in chemical research and data.
Strategy Settings
Precursor scoring | Relevance Heuristic |
---|---|
Min. plausibility | 0.01 |
Model | Template_relevance |
Template Set | Pistachio/Bkms_metabolic/Pistachio_ringbreaker/Reaxys/Reaxys_biocatalysis |
Top-N result to add to graph | 6 |
Feasible Synthetic Routes
Disclaimer and Information on In-Vitro Research Products
Please be aware that all articles and product information presented on BenchChem are intended solely for informational purposes. The products available for purchase on BenchChem are specifically designed for in-vitro studies, which are conducted outside of living organisms. In-vitro studies, derived from the Latin term "in glass," involve experiments performed in controlled laboratory settings using cells or tissues. It is important to note that these products are not categorized as medicines or drugs, and they have not received approval from the FDA for the prevention, treatment, or cure of any medical condition, ailment, or disease. We must emphasize that any form of bodily introduction of these products into humans or animals is strictly prohibited by law. It is essential to adhere to these guidelines to ensure compliance with legal and ethical standards in research and experimentation.