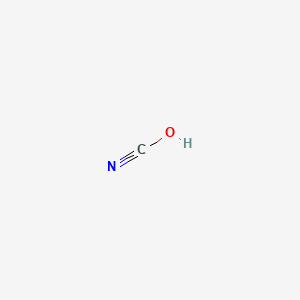
Cyanic acid
Overview
Description
Cyanate, also known as Cyanic acid or [C(N)OH], is classified as a cyanate. Cyanates are organic compounds containing the cyanate functional group with the formula [OCN]-. Cyanate is considered a soluble (in water), acidic compound. Cyanate may be a unique E. coli metabolite. The cyanate ion is an anion consisting of one oxygen atom, one carbon atom, and one nitrogen atom, [OCN], in that order. The cyanate ion possesses 1 unit of negative charge, borne mainly by the nitrogen atom. In organic compounds the cyanate group is a functional group. ; The cyanate ion is an ambident nucleophile in nucleophilic substitution because it can react to form an alkyl cyanate R-OCN (exception) or an alkyl isocyanate R-NCO (rule). Aryl cyanates (C6H5OCN) can be formed by a reaction of phenol with cyanogen chloride (ClCN) in the presence of a base. The cyanate ion is relatively non-toxic in comparison with cyanides. Use of this fact is made in cyanide decontamination processes where a permanganate oxidation converts toxic cyanide to safer cyanate. Cyanate can be decomposed by the enzyme cyanate lyase (or cyanase), which is found in bacteria and plants. In particular cyanate can be decomposed to carbamate (ammonia) and carbon dioxide. Alternately the same enzyme can be used to synthesize cyanate using carbamate and carbon dioxide.
This compound is a one-carbon compound and a pseudohalogen oxoacid. It is a conjugate acid of a cyanate. It is a tautomer of an isothis compound.
Properties
CAS No. |
420-05-3 |
---|---|
Molecular Formula |
CHNO |
Molecular Weight |
43.025 g/mol |
IUPAC Name |
cyanic acid |
InChI |
InChI=1S/CHNO/c2-1-3/h3H |
InChI Key |
XLJMAIOERFSOGZ-UHFFFAOYSA-N |
SMILES |
C(#N)O |
Canonical SMILES |
C(#N)O |
melting_point |
-86.0 °C |
420-05-3 71000-82-3 |
|
physical_description |
Solid |
vapor_pressure |
812.46 mmHg |
Origin of Product |
United States |
Synthesis routes and methods
Procedure details
Retrosynthesis Analysis
AI-Powered Synthesis Planning: Our tool employs the Template_relevance Pistachio, Template_relevance Bkms_metabolic, Template_relevance Pistachio_ringbreaker, Template_relevance Reaxys, Template_relevance Reaxys_biocatalysis model, leveraging a vast database of chemical reactions to predict feasible synthetic routes.
One-Step Synthesis Focus: Specifically designed for one-step synthesis, it provides concise and direct routes for your target compounds, streamlining the synthesis process.
Accurate Predictions: Utilizing the extensive PISTACHIO, BKMS_METABOLIC, PISTACHIO_RINGBREAKER, REAXYS, REAXYS_BIOCATALYSIS database, our tool offers high-accuracy predictions, reflecting the latest in chemical research and data.
Strategy Settings
Precursor scoring | Relevance Heuristic |
---|---|
Min. plausibility | 0.01 |
Model | Template_relevance |
Template Set | Pistachio/Bkms_metabolic/Pistachio_ringbreaker/Reaxys/Reaxys_biocatalysis |
Top-N result to add to graph | 6 |
Feasible Synthetic Routes
Disclaimer and Information on In-Vitro Research Products
Please be aware that all articles and product information presented on BenchChem are intended solely for informational purposes. The products available for purchase on BenchChem are specifically designed for in-vitro studies, which are conducted outside of living organisms. In-vitro studies, derived from the Latin term "in glass," involve experiments performed in controlled laboratory settings using cells or tissues. It is important to note that these products are not categorized as medicines or drugs, and they have not received approval from the FDA for the prevention, treatment, or cure of any medical condition, ailment, or disease. We must emphasize that any form of bodily introduction of these products into humans or animals is strictly prohibited by law. It is essential to adhere to these guidelines to ensure compliance with legal and ethical standards in research and experimentation.