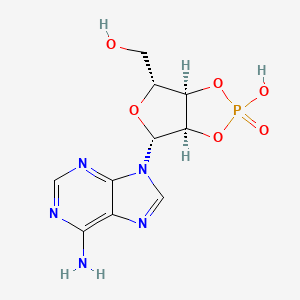
2',3'-Cyclic AMP
Overview
Description
2’,3’-Cyclic Adenosine Monophosphate is a cyclic nucleotide derived from adenosine triphosphate. It plays a crucial role as a second messenger in various biological processes, including intracellular signal transduction. This compound is involved in the regulation of metabolism and gene expression in all life forms .
Mechanism of Action
Target of Action
2’,3’-Cyclic AMP, also known as cyclic adenosine monophosphate (cAMP), is a crucial second messenger in numerous signal transduction pathways . It primarily targets protein kinases, ion channels such as the HCN channels, and a few other cyclic nucleotide-binding proteins such as Epac1 and RAPGEF2 . These targets play vital roles in various biological processes, including cell growth and differentiation, gene transcription, and protein expression .
Mode of Action
The interaction of 2’,3’-Cyclic AMP with its targets leads to significant changes within the cell. For instance, cAMP binds to protein kinase A, which then catalyzes the transfer of phosphate from ATP to a serine residue on a second enzyme, phosphorylase kinase . This process triggers a series of biochemical reactions that result in several physiological effects .
Biochemical Pathways
2’,3’-Cyclic AMP is involved in the cAMP-dependent pathway . It is synthesized from ATP by adenylate cyclase located on the inner side of the plasma membrane . The adenylate cyclase is activated by a range of signaling molecules through the activation of adenylate cyclase stimulatory G-protein-coupled receptors . The decomposition of cAMP into AMP is catalyzed by the enzyme phosphodiesterase . This pathway plays a key role in intracellular signal transduction, transferring into cells the effects of hormones like glucagon and adrenaline, which cannot pass through the plasma membrane .
Pharmacokinetics
It is known that camp is short-lived because it is rapidly hydrolyzed by camp phosphodiesterase, yielding 5´-amp . This rapid degradation could impact the bioavailability of cAMP within the cell.
Result of Action
The action of 2’,3’-Cyclic AMP leads to a variety of molecular and cellular effects. It is used for intracellular signal transduction, transferring into cells the effects of hormones like glucagon and adrenaline . It is also involved in the activation of protein kinases . In addition, cAMP binds to and regulates the function of ion channels such as the HCN channels and a few other cyclic nucleotide-binding proteins such as Epac1 and RAPGEF2 .
Action Environment
The action, efficacy, and stability of 2’,3’-Cyclic AMP can be influenced by various environmental factors. For instance, the presence of certain signaling molecules can activate adenylate cyclase, leading to the synthesis of cAMP . Additionally, the action of cAMP can be modulated by the presence of other cellular components, such as G-protein-coupled receptors
Preparation Methods
Synthetic Routes and Reaction Conditions: 2’,3’-Cyclic Adenosine Monophosphate can be synthesized chemically or biocatalytically. The chemical synthesis involves the cyclization of adenosine monophosphate using specific catalysts and reaction conditions. this method often results in low yields and requires the use of organic solvents .
Industrial Production Methods: Biocatalytic synthesis is preferred for industrial production due to its higher efficiency and environmental friendliness. This method utilizes enzymes such as cyclic guanosine monophosphate–adenosine monophosphate synthase, which catalyzes the formation of 2’,3’-Cyclic Adenosine Monophosphate from adenosine triphosphate in the presence of divalent cations .
Chemical Reactions Analysis
Types of Reactions: 2’,3’-Cyclic Adenosine Monophosphate undergoes various chemical reactions, including hydrolysis, phosphorylation, and cyclization.
Common Reagents and Conditions:
Hydrolysis: Catalyzed by phosphodiesterases, resulting in the formation of adenosine monophosphate.
Phosphorylation: Involves the addition of phosphate groups, often mediated by protein kinases.
Cyclization: Catalyzed by adenylate cyclase, converting adenosine triphosphate to 2’,3’-Cyclic Adenosine Monophosphate.
Major Products:
Hydrolysis: Adenosine monophosphate.
Phosphorylation: Various phosphorylated intermediates.
Cyclization: 2’,3’-Cyclic Adenosine Monophosphate.
Scientific Research Applications
2’,3’-Cyclic Adenosine Monophosphate has extensive applications in scientific research:
Chemistry: Used as a model compound to study cyclic nucleotide signaling pathways.
Industry: Utilized in the production of pharmaceuticals and as a research tool in biotechnology.
Comparison with Similar Compounds
3’,5’-Cyclic Adenosine Monophosphate: Another cyclic nucleotide with similar signaling functions but different structural properties.
2’,3’-Cyclic Guanosine Monophosphate: Shares similar signaling roles but involves guanosine instead of adenosine.
Uniqueness: 2’,3’-Cyclic Adenosine Monophosphate is unique due to its specific role in certain signaling pathways and its distinct structural configuration, which influences its interaction with various enzymes and receptors .
Properties
IUPAC Name |
[4-(6-aminopurin-9-yl)-2-hydroxy-2-oxo-3a,4,6,6a-tetrahydrofuro[3,4-d][1,3,2]dioxaphosphol-6-yl]methanol | |
---|---|---|
Details | Computed by Lexichem TK 2.7.0 (PubChem release 2021.05.07) | |
Source | PubChem | |
URL | https://pubchem.ncbi.nlm.nih.gov | |
Description | Data deposited in or computed by PubChem | |
InChI |
InChI=1S/C10H12N5O6P/c11-8-5-9(13-2-12-8)15(3-14-5)10-7-6(4(1-16)19-10)20-22(17,18)21-7/h2-4,6-7,10,16H,1H2,(H,17,18)(H2,11,12,13) | |
Details | Computed by InChI 1.0.6 (PubChem release 2021.05.07) | |
Source | PubChem | |
URL | https://pubchem.ncbi.nlm.nih.gov | |
Description | Data deposited in or computed by PubChem | |
InChI Key |
KMYWVDDIPVNLME-UHFFFAOYSA-N | |
Details | Computed by InChI 1.0.6 (PubChem release 2021.05.07) | |
Source | PubChem | |
URL | https://pubchem.ncbi.nlm.nih.gov | |
Description | Data deposited in or computed by PubChem | |
Canonical SMILES |
C1=NC(=C2C(=N1)N(C=N2)C3C4C(C(O3)CO)OP(=O)(O4)O)N | |
Details | Computed by OEChem 2.3.0 (PubChem release 2021.05.07) | |
Source | PubChem | |
URL | https://pubchem.ncbi.nlm.nih.gov | |
Description | Data deposited in or computed by PubChem | |
Molecular Formula |
C10H12N5O6P | |
Details | Computed by PubChem 2.1 (PubChem release 2021.05.07) | |
Source | PubChem | |
URL | https://pubchem.ncbi.nlm.nih.gov | |
Description | Data deposited in or computed by PubChem | |
Molecular Weight |
329.21 g/mol | |
Details | Computed by PubChem 2.1 (PubChem release 2021.05.07) | |
Source | PubChem | |
URL | https://pubchem.ncbi.nlm.nih.gov | |
Description | Data deposited in or computed by PubChem | |
Physical Description |
Solid | |
Record name | Adenosine 2',3'-cyclic phosphate | |
Source | Human Metabolome Database (HMDB) | |
URL | http://www.hmdb.ca/metabolites/HMDB0011616 | |
Description | The Human Metabolome Database (HMDB) is a freely available electronic database containing detailed information about small molecule metabolites found in the human body. | |
Explanation | HMDB is offered to the public as a freely available resource. Use and re-distribution of the data, in whole or in part, for commercial purposes requires explicit permission of the authors and explicit acknowledgment of the source material (HMDB) and the original publication (see the HMDB citing page). We ask that users who download significant portions of the database cite the HMDB paper in any resulting publications. | |
CAS No. |
37063-35-7, 634-01-5 | |
Record name | Adenosine sodio-2',3'-phosphate hydrate | |
Source | European Chemicals Agency (ECHA) | |
URL | https://echa.europa.eu/substance-information/-/substanceinfo/100.048.465 | |
Description | The European Chemicals Agency (ECHA) is an agency of the European Union which is the driving force among regulatory authorities in implementing the EU's groundbreaking chemicals legislation for the benefit of human health and the environment as well as for innovation and competitiveness. | |
Explanation | Use of the information, documents and data from the ECHA website is subject to the terms and conditions of this Legal Notice, and subject to other binding limitations provided for under applicable law, the information, documents and data made available on the ECHA website may be reproduced, distributed and/or used, totally or in part, for non-commercial purposes provided that ECHA is acknowledged as the source: "Source: European Chemicals Agency, http://echa.europa.eu/". Such acknowledgement must be included in each copy of the material. ECHA permits and encourages organisations and individuals to create links to the ECHA website under the following cumulative conditions: Links can only be made to webpages that provide a link to the Legal Notice page. | |
Record name | Adenosine 2',3'-cyclic phosphate | |
Source | Human Metabolome Database (HMDB) | |
URL | http://www.hmdb.ca/metabolites/HMDB0011616 | |
Description | The Human Metabolome Database (HMDB) is a freely available electronic database containing detailed information about small molecule metabolites found in the human body. | |
Explanation | HMDB is offered to the public as a freely available resource. Use and re-distribution of the data, in whole or in part, for commercial purposes requires explicit permission of the authors and explicit acknowledgment of the source material (HMDB) and the original publication (see the HMDB citing page). We ask that users who download significant portions of the database cite the HMDB paper in any resulting publications. | |
Retrosynthesis Analysis
AI-Powered Synthesis Planning: Our tool employs the Template_relevance Pistachio, Template_relevance Bkms_metabolic, Template_relevance Pistachio_ringbreaker, Template_relevance Reaxys, Template_relevance Reaxys_biocatalysis model, leveraging a vast database of chemical reactions to predict feasible synthetic routes.
One-Step Synthesis Focus: Specifically designed for one-step synthesis, it provides concise and direct routes for your target compounds, streamlining the synthesis process.
Accurate Predictions: Utilizing the extensive PISTACHIO, BKMS_METABOLIC, PISTACHIO_RINGBREAKER, REAXYS, REAXYS_BIOCATALYSIS database, our tool offers high-accuracy predictions, reflecting the latest in chemical research and data.
Strategy Settings
Precursor scoring | Relevance Heuristic |
---|---|
Min. plausibility | 0.01 |
Model | Template_relevance |
Template Set | Pistachio/Bkms_metabolic/Pistachio_ringbreaker/Reaxys/Reaxys_biocatalysis |
Top-N result to add to graph | 6 |
Feasible Synthetic Routes
Disclaimer and Information on In-Vitro Research Products
Please be aware that all articles and product information presented on BenchChem are intended solely for informational purposes. The products available for purchase on BenchChem are specifically designed for in-vitro studies, which are conducted outside of living organisms. In-vitro studies, derived from the Latin term "in glass," involve experiments performed in controlled laboratory settings using cells or tissues. It is important to note that these products are not categorized as medicines or drugs, and they have not received approval from the FDA for the prevention, treatment, or cure of any medical condition, ailment, or disease. We must emphasize that any form of bodily introduction of these products into humans or animals is strictly prohibited by law. It is essential to adhere to these guidelines to ensure compliance with legal and ethical standards in research and experimentation.