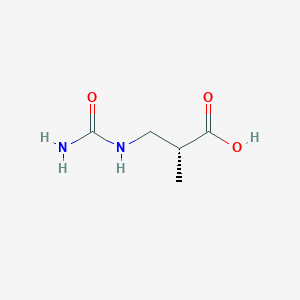
(R)-3-Ureidoisobutyrate
- Click on QUICK INQUIRY to receive a quote from our team of experts.
- With the quality product at a COMPETITIVE price, you can focus more on your research.
Overview
Description
®-3-Ureidoisobutyrate is a chemical compound that has garnered interest in various scientific fields due to its unique structure and properties. This compound is characterized by the presence of a ureido group attached to an isobutyrate backbone, which imparts specific chemical reactivity and potential biological activity.
Preparation Methods
Synthetic Routes and Reaction Conditions
The synthesis of ®-3-Ureidoisobutyrate typically involves the reaction of isobutyric acid derivatives with urea under controlled conditions. One common method includes the use of isobutyric acid chloride, which reacts with urea in the presence of a base such as triethylamine. The reaction is usually carried out in an organic solvent like dichloromethane at low temperatures to ensure high yield and purity.
Industrial Production Methods
On an industrial scale, the production of ®-3-Ureidoisobutyrate may involve continuous flow reactors to maintain consistent reaction conditions and improve efficiency. The use of catalysts and optimized reaction parameters can further enhance the yield and reduce production costs.
Chemical Reactions Analysis
Types of Reactions
®-3-Ureidoisobutyrate can undergo various chemical reactions, including:
Oxidation: The compound can be oxidized using agents like potassium permanganate or hydrogen peroxide, leading to the formation of corresponding oxidized products.
Reduction: Reduction reactions can be carried out using reducing agents such as lithium aluminum hydride, resulting in the formation of amine derivatives.
Substitution: Nucleophilic substitution reactions can occur at the ureido group, where nucleophiles like amines or thiols replace the existing substituents.
Common Reagents and Conditions
Oxidation: Potassium permanganate in an aqueous medium at room temperature.
Reduction: Lithium aluminum hydride in anhydrous ether at low temperatures.
Substitution: Amines or thiols in the presence of a base like sodium hydroxide in an organic solvent.
Major Products Formed
Oxidation: Oxidized derivatives such as carboxylic acids or ketones.
Reduction: Amine derivatives.
Substitution: Ureido derivatives with different substituents.
Scientific Research Applications
Chemistry
In chemistry, ®-3-Ureidoisobutyrate is used as a building block for the synthesis of more complex molecules. Its unique reactivity makes it valuable in the development of new synthetic methodologies and the study of reaction mechanisms.
Biology
In biological research, ®-3-Ureidoisobutyrate is investigated for its potential role in metabolic pathways. It can serve as a substrate or inhibitor in enzymatic reactions, providing insights into enzyme function and regulation.
Medicine
In medicine, ®-3-Ureidoisobutyrate is explored for its potential therapeutic applications. Its derivatives may exhibit biological activity that could be harnessed for the development of new drugs targeting specific diseases.
Industry
In the industrial sector, ®-3-Ureidoisobutyrate is used in the production of specialty chemicals and materials. Its unique properties make it suitable for applications in polymer synthesis and the development of advanced materials.
Mechanism of Action
The mechanism of action of ®-3-Ureidoisobutyrate involves its interaction with specific molecular targets, such as enzymes or receptors. The ureido group can form hydrogen bonds and other interactions with active sites, modulating the activity of the target molecules. This interaction can lead to changes in metabolic pathways or cellular processes, contributing to the compound’s biological effects.
Comparison with Similar Compounds
Similar Compounds
(S)-3-Ureidoisobutyrate: The enantiomer of ®-3-Ureidoisobutyrate, which may exhibit different biological activity due to its stereochemistry.
N-Carbamoyl-β-alanine: A structurally similar compound with a carbamoyl group instead of a ureido group.
Ureidoisobutyric acid: A related compound with a similar backbone but different functional groups.
Uniqueness
®-3-Ureidoisobutyrate is unique due to its specific stereochemistry and the presence of the ureido group, which imparts distinct chemical reactivity and potential biological activity. Its ability to undergo various chemical reactions and interact with molecular targets makes it a valuable compound in scientific research and industrial applications.
Properties
Molecular Formula |
C5H10N2O3 |
---|---|
Molecular Weight |
146.14 g/mol |
IUPAC Name |
(2R)-3-(carbamoylamino)-2-methylpropanoic acid |
InChI |
InChI=1S/C5H10N2O3/c1-3(4(8)9)2-7-5(6)10/h3H,2H2,1H3,(H,8,9)(H3,6,7,10)/t3-/m1/s1 |
InChI Key |
PHENTZNALBMCQD-GSVOUGTGSA-N |
Isomeric SMILES |
C[C@H](CNC(=O)N)C(=O)O |
Canonical SMILES |
CC(CNC(=O)N)C(=O)O |
Origin of Product |
United States |
Disclaimer and Information on In-Vitro Research Products
Please be aware that all articles and product information presented on BenchChem are intended solely for informational purposes. The products available for purchase on BenchChem are specifically designed for in-vitro studies, which are conducted outside of living organisms. In-vitro studies, derived from the Latin term "in glass," involve experiments performed in controlled laboratory settings using cells or tissues. It is important to note that these products are not categorized as medicines or drugs, and they have not received approval from the FDA for the prevention, treatment, or cure of any medical condition, ailment, or disease. We must emphasize that any form of bodily introduction of these products into humans or animals is strictly prohibited by law. It is essential to adhere to these guidelines to ensure compliance with legal and ethical standards in research and experimentation.