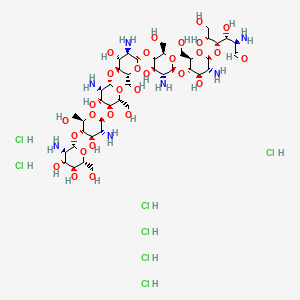
Chitoheptaose (heptahydrochloride)
- Click on QUICK INQUIRY to receive a quote from our team of experts.
- With the quality product at a COMPETITIVE price, you can focus more on your research.
Overview
Description
Chitoheptaose (heptahydrochloride) is a chitosan oligosaccharide, which is a derivative of chitin. It is composed of seven D-glucosamine units linked by β(1→4) glycosidic bonds. This compound is known for its antioxidant, anti-inflammatory, antiapoptotic, and cardioprotective properties .
Preparation Methods
Synthetic Routes and Reaction Conditions: Chitoheptaose (heptahydrochloride) can be synthesized through the partial hydrolysis of chitosan, which is derived from chitin. The hydrolysis process involves the use of acids or enzymes to break down chitosan into smaller oligosaccharides. The resulting chitoheptaose is then treated with hydrochloric acid to form the heptahydrochloride salt .
Industrial Production Methods: Industrial production of chitoheptaose (heptahydrochloride) involves modern separation technologies such as integrated membrane separation, adsorption, distribution, gel exclusion, and ion exchange. These methods ensure high purity and yield of the compound .
Chemical Reactions Analysis
Types of Reactions: Chitoheptaose (heptahydrochloride) undergoes various chemical reactions, including:
Oxidation: It can be oxidized to form corresponding aldehydes or carboxylic acids.
Reduction: Reduction reactions can convert it into its corresponding alcohols.
Substitution: It can undergo substitution reactions where functional groups are replaced by other groups.
Common Reagents and Conditions:
Oxidation: Common oxidizing agents include hydrogen peroxide and potassium permanganate.
Reduction: Reducing agents such as sodium borohydride and lithium aluminum hydride are used.
Substitution: Conditions vary depending on the substituent being introduced, but common reagents include halogens and alkylating agents.
Major Products: The major products formed from these reactions include various derivatives of chitoheptaose, such as its oxidized, reduced, or substituted forms .
Scientific Research Applications
Chitoheptaose (heptahydrochloride) has a wide range of applications in scientific research:
Chemistry: It is used as a reagent in various chemical reactions and as a precursor for the synthesis of other compounds.
Biology: It has been shown to enhance the growth and photosynthesis parameters of wheat seedlings.
Mechanism of Action
Chitoheptaose (heptahydrochloride) exerts its effects through several molecular pathways:
Antioxidant Activity: It scavenges reactive oxygen species (ROS) and reactive nitrogen species (RNS), reducing oxidative stress.
Anti-inflammatory Activity: It reduces the levels of pro-inflammatory cytokines such as interleukin-1β, interleukin-17A, and interferon-γ, while increasing the level of anti-inflammatory cytokine interleukin-10.
Antiapoptotic Activity: It inhibits apoptotic pathways by modulating the expression of apoptotic factors such as caspase 3, BAX, and BCL-2.
Comparison with Similar Compounds
Chitoheptaose (heptahydrochloride) is unique among chitosan oligosaccharides due to its specific degree of polymerization and its heptahydrochloride form. Similar compounds include:
- Chitotetraose tetrahydrochloride
- Chitobiose dihydrochloride
- Chitopentaose pentahydrochloride
- Chitohexaose hexahydrochloride
- Chitotriose trihydrochloride
These compounds share similar properties but differ in their degree of polymerization and specific applications .
Properties
Molecular Formula |
C42H86Cl7N7O29 |
---|---|
Molecular Weight |
1401.3 g/mol |
IUPAC Name |
(2R,3R,4S,5R)-2-amino-4-[(2S,3R,4R,5S,6R)-3-amino-5-[(2S,3R,4R,5S,6R)-3-amino-5-[(2S,3R,4R,5S,6R)-3-amino-5-[(2S,3R,4R,5S,6R)-3-amino-5-[(2S,3R,4R,5S,6R)-3-amino-5-[(2S,3R,4R,5S,6R)-3-amino-4,5-dihydroxy-6-(hydroxymethyl)oxan-2-yl]oxy-4-hydroxy-6-(hydroxymethyl)oxan-2-yl]oxy-4-hydroxy-6-(hydroxymethyl)oxan-2-yl]oxy-4-hydroxy-6-(hydroxymethyl)oxan-2-yl]oxy-4-hydroxy-6-(hydroxymethyl)oxan-2-yl]oxy-4-hydroxy-6-(hydroxymethyl)oxan-2-yl]oxy-3,5,6-trihydroxyhexanal;heptahydrochloride |
InChI |
InChI=1S/C42H79N7O29.7ClH/c43-9(1-50)23(59)31(10(58)2-51)73-38-18(45)26(62)33(12(4-53)68-38)75-40-20(47)28(64)35(14(6-55)70-40)77-42-22(49)30(66)36(16(8-57)72-42)78-41-21(48)29(65)34(15(7-56)71-41)76-39-19(46)27(63)32(13(5-54)69-39)74-37-17(44)25(61)24(60)11(3-52)67-37;;;;;;;/h1,9-42,51-66H,2-8,43-49H2;7*1H/t9-,10+,11+,12+,13+,14+,15+,16+,17+,18+,19+,20+,21+,22+,23+,24+,25+,26+,27+,28+,29+,30+,31+,32+,33+,34+,35+,36+,37-,38-,39-,40-,41-,42-;;;;;;;/m0......./s1 |
InChI Key |
LZTOWEFZDLXZFB-KWMOADEZSA-N |
Isomeric SMILES |
C([C@@H]1[C@H]([C@@H]([C@H]([C@@H](O1)O[C@@H]2[C@H](O[C@H]([C@@H]([C@H]2O)N)O[C@@H]3[C@H](O[C@H]([C@@H]([C@H]3O)N)O[C@@H]4[C@H](O[C@H]([C@@H]([C@H]4O)N)O[C@@H]5[C@H](O[C@H]([C@@H]([C@H]5O)N)O[C@@H]6[C@H](O[C@H]([C@@H]([C@H]6O)N)O[C@H]([C@@H](CO)O)[C@@H]([C@H](C=O)N)O)CO)CO)CO)CO)CO)N)O)O)O.Cl.Cl.Cl.Cl.Cl.Cl.Cl |
Canonical SMILES |
C(C1C(C(C(C(O1)OC2C(OC(C(C2O)N)OC3C(OC(C(C3O)N)OC4C(OC(C(C4O)N)OC5C(OC(C(C5O)N)OC6C(OC(C(C6O)N)OC(C(CO)O)C(C(C=O)N)O)CO)CO)CO)CO)CO)N)O)O)O.Cl.Cl.Cl.Cl.Cl.Cl.Cl |
Origin of Product |
United States |
Disclaimer and Information on In-Vitro Research Products
Please be aware that all articles and product information presented on BenchChem are intended solely for informational purposes. The products available for purchase on BenchChem are specifically designed for in-vitro studies, which are conducted outside of living organisms. In-vitro studies, derived from the Latin term "in glass," involve experiments performed in controlled laboratory settings using cells or tissues. It is important to note that these products are not categorized as medicines or drugs, and they have not received approval from the FDA for the prevention, treatment, or cure of any medical condition, ailment, or disease. We must emphasize that any form of bodily introduction of these products into humans or animals is strictly prohibited by law. It is essential to adhere to these guidelines to ensure compliance with legal and ethical standards in research and experimentation.