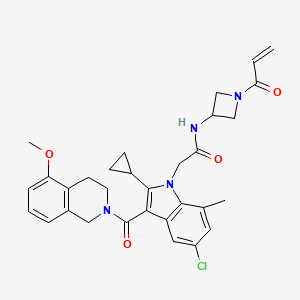
K-Ras G12C-IN-4
- Click on QUICK INQUIRY to receive a quote from our team of experts.
- With the quality product at a COMPETITIVE price, you can focus more on your research.
Overview
Description
K-Ras G12C-IN-4 is a small molecule inhibitor specifically designed to target the Kirsten rat sarcoma viral oncogene homolog (KRAS) protein with a glycine-to-cysteine mutation at codon 12 (G12C). This mutation is commonly found in various cancers, including non-small cell lung cancer, colorectal cancer, and pancreatic cancer. The compound binds covalently to the mutant cysteine residue, thereby inhibiting the oncogenic activity of KRAS G12C and blocking downstream signaling pathways that promote cancer cell proliferation and survival .
Preparation Methods
Synthetic Routes and Reaction Conditions
The synthesis of K-Ras G12C-IN-4 involves multiple steps, starting with the preparation of key intermediates. The synthetic route typically includes:
Formation of the core structure: This involves the construction of the central scaffold through a series of condensation and cyclization reactions.
Functionalization: Introduction of functional groups that enhance binding affinity and specificity to the KRAS G12C mutant.
Purification: The final compound is purified using techniques such as column chromatography and recrystallization to achieve high purity.
Industrial Production Methods
Industrial production of this compound follows similar synthetic routes but on a larger scale. This involves optimization of reaction conditions to maximize yield and minimize impurities. Techniques such as high-performance liquid chromatography (HPLC) are employed for large-scale purification .
Chemical Reactions Analysis
Types of Reactions
K-Ras G12C-IN-4 primarily undergoes covalent binding reactions with the cysteine residue of the KRAS G12C mutant. This covalent modification is crucial for its inhibitory activity. Additionally, the compound may undergo metabolic reactions in the body, leading to the formation of metabolites .
Common Reagents and Conditions
Reagents: Common reagents used in the synthesis and reactions of this compound include organic solvents (e.g., dimethyl sulfoxide), bases (e.g., sodium hydroxide), and acids (e.g., hydrochloric acid).
Major Products
The major product of the reaction between this compound and the KRAS G12C mutant is the covalently modified KRAS protein, which is rendered inactive. Metabolic reactions may produce various metabolites, which are typically analyzed using mass spectrometry .
Scientific Research Applications
K-Ras G12C-IN-4 has a wide range of applications in scientific research, particularly in the fields of cancer biology and drug development:
Cancer Research: It is used to study the role of KRAS G12C in cancer progression and to evaluate the efficacy of KRAS-targeted therapies.
Drug Development: The compound serves as a lead molecule for the development of new KRAS inhibitors with improved potency and selectivity.
Biological Studies: Researchers use this compound to investigate the downstream signaling pathways activated by KRAS G12C and to identify potential biomarkers for cancer diagnosis and treatment.
Mechanism of Action
K-Ras G12C-IN-4 exerts its effects by covalently binding to the cysteine residue at codon 12 of the KRAS protein. This binding locks the protein in its inactive GDP-bound state, preventing the exchange of GDP for GTP and thereby inhibiting KRAS activation. As a result, downstream signaling pathways such as the RAF-MEK-ERK and PI3K-AKT pathways are blocked, leading to reduced cancer cell proliferation and survival .
Comparison with Similar Compounds
K-Ras G12C-IN-4 is part of a class of KRAS G12C inhibitors that includes compounds such as sotorasib and adagrasib. Compared to these inhibitors, this compound may offer unique advantages in terms of binding affinity, selectivity, and pharmacokinetic properties. Similar compounds include:
Sotorasib: The first FDA-approved KRAS G12C inhibitor, known for its efficacy in non-small cell lung cancer.
Adagrasib: Another KRAS G12C inhibitor with promising clinical trial results.
This compound’s unique binding mechanism and potential for combination therapy make it a valuable tool in cancer research and treatment development.
Properties
Molecular Formula |
C31H33ClN4O4 |
---|---|
Molecular Weight |
561.1 g/mol |
IUPAC Name |
2-[5-chloro-2-cyclopropyl-3-(5-methoxy-3,4-dihydro-1H-isoquinoline-2-carbonyl)-7-methylindol-1-yl]-N-(1-prop-2-enoylazetidin-3-yl)acetamide |
InChI |
InChI=1S/C31H33ClN4O4/c1-4-27(38)35-15-22(16-35)33-26(37)17-36-29-18(2)12-21(32)13-24(29)28(30(36)19-8-9-19)31(39)34-11-10-23-20(14-34)6-5-7-25(23)40-3/h4-7,12-13,19,22H,1,8-11,14-17H2,2-3H3,(H,33,37) |
InChI Key |
JESBUJUEMLEHDQ-UHFFFAOYSA-N |
Canonical SMILES |
CC1=CC(=CC2=C1N(C(=C2C(=O)N3CCC4=C(C3)C=CC=C4OC)C5CC5)CC(=O)NC6CN(C6)C(=O)C=C)Cl |
Origin of Product |
United States |
Disclaimer and Information on In-Vitro Research Products
Please be aware that all articles and product information presented on BenchChem are intended solely for informational purposes. The products available for purchase on BenchChem are specifically designed for in-vitro studies, which are conducted outside of living organisms. In-vitro studies, derived from the Latin term "in glass," involve experiments performed in controlled laboratory settings using cells or tissues. It is important to note that these products are not categorized as medicines or drugs, and they have not received approval from the FDA for the prevention, treatment, or cure of any medical condition, ailment, or disease. We must emphasize that any form of bodily introduction of these products into humans or animals is strictly prohibited by law. It is essential to adhere to these guidelines to ensure compliance with legal and ethical standards in research and experimentation.