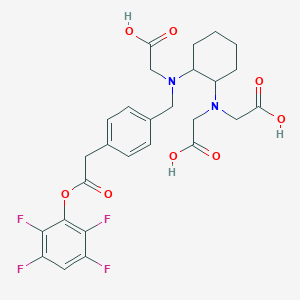
(+/-)-H3Resca-tfp
- Click on QUICK INQUIRY to receive a quote from our team of experts.
- With the quality product at a COMPETITIVE price, you can focus more on your research.
Overview
Description
(+/-)-H3Resca-tfp is a chiral compound with significant interest in various scientific fields due to its unique chemical properties and potential applications. The compound is known for its complex structure and the presence of multiple functional groups, making it a versatile molecule for research and industrial purposes.
Preparation Methods
Synthetic Routes and Reaction Conditions
The synthesis of (+/-)-H3Resca-tfp involves multiple steps, typically starting with the preparation of the core structure followed by the introduction of specific functional groups. The reaction conditions often require precise control of temperature, pH, and the use of specific catalysts to ensure the desired stereochemistry is achieved.
Industrial Production Methods
Industrial production of this compound generally involves large-scale synthesis using optimized reaction conditions to maximize yield and purity. This often includes the use of continuous flow reactors and advanced purification techniques such as chromatography and crystallization.
Chemical Reactions Analysis
Types of Reactions
(+/-)-H3Resca-tfp undergoes various types of chemical reactions, including:
Oxidation: The compound can be oxidized to form different oxidation states, often using reagents like potassium permanganate or chromium trioxide.
Reduction: Reduction reactions can be carried out using hydrogen gas in the presence of a palladium catalyst.
Substitution: Nucleophilic substitution reactions are common, where functional groups are replaced by nucleophiles such as halides or amines.
Common Reagents and Conditions
Oxidation: Potassium permanganate, chromium trioxide, and other strong oxidizing agents.
Reduction: Hydrogen gas with palladium catalyst, lithium aluminum hydride.
Substitution: Halides, amines, and other nucleophiles under basic or acidic conditions.
Major Products Formed
The major products formed from these reactions depend on the specific functional groups involved and the reaction conditions. For example, oxidation may yield ketones or carboxylic acids, while reduction can produce alcohols or alkanes.
Scientific Research Applications
(+/-)-H3Resca-tfp has a wide range of applications in scientific research, including:
Chemistry: Used as a building block for the synthesis of more complex molecules.
Biology: Studied for its potential biological activity and interactions with biomolecules.
Medicine: Investigated for its potential therapeutic properties, including anti-inflammatory and anticancer activities.
Industry: Utilized in the production of specialty chemicals and materials.
Mechanism of Action
The mechanism of action of (+/-)-H3Resca-tfp involves its interaction with specific molecular targets, such as enzymes or receptors. The compound can modulate the activity of these targets, leading to various biological effects. The pathways involved often include signal transduction cascades and metabolic processes.
Comparison with Similar Compounds
Similar Compounds
(+/-)-H3Resca-tfp: shares similarities with other chiral compounds such as this compound analogs and derivatives.
Unique Features:
List of Similar Compounds
- This compound analogs
- This compound derivatives
- Other chiral compounds with similar functional groups
Properties
Molecular Formula |
C27H28F4N2O8 |
---|---|
Molecular Weight |
584.5 g/mol |
IUPAC Name |
2-[[2-[bis(carboxymethyl)amino]cyclohexyl]-[[4-[2-oxo-2-(2,3,5,6-tetrafluorophenoxy)ethyl]phenyl]methyl]amino]acetic acid |
InChI |
InChI=1S/C27H28F4N2O8/c28-17-10-18(29)26(31)27(25(17)30)41-24(40)9-15-5-7-16(8-6-15)11-32(12-21(34)35)19-3-1-2-4-20(19)33(13-22(36)37)14-23(38)39/h5-8,10,19-20H,1-4,9,11-14H2,(H,34,35)(H,36,37)(H,38,39) |
InChI Key |
GKFBSFKLJKSHSZ-UHFFFAOYSA-N |
Canonical SMILES |
C1CCC(C(C1)N(CC2=CC=C(C=C2)CC(=O)OC3=C(C(=CC(=C3F)F)F)F)CC(=O)O)N(CC(=O)O)CC(=O)O |
Origin of Product |
United States |
Disclaimer and Information on In-Vitro Research Products
Please be aware that all articles and product information presented on BenchChem are intended solely for informational purposes. The products available for purchase on BenchChem are specifically designed for in-vitro studies, which are conducted outside of living organisms. In-vitro studies, derived from the Latin term "in glass," involve experiments performed in controlled laboratory settings using cells or tissues. It is important to note that these products are not categorized as medicines or drugs, and they have not received approval from the FDA for the prevention, treatment, or cure of any medical condition, ailment, or disease. We must emphasize that any form of bodily introduction of these products into humans or animals is strictly prohibited by law. It is essential to adhere to these guidelines to ensure compliance with legal and ethical standards in research and experimentation.