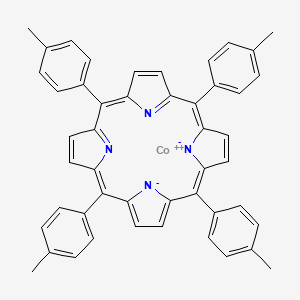
Cobalt(2+);5,10,15,20-tetrakis(4-methylphenyl)porphyrin-22,23-diide
- Click on QUICK INQUIRY to receive a quote from our team of experts.
- With the quality product at a COMPETITIVE price, you can focus more on your research.
Overview
Description
Cobalt(2+);5,10,15,20-tetrakis(4-methylphenyl)porphyrin-22,23-diide (hereafter referred to as Co-TMPP²⁻) is a metalloporphyrin complex characterized by a cobalt(II) ion coordinated within a porphyrin macrocycle substituted with four 4-methylphenyl groups at the meso positions. The methyl substituents enhance hydrophobicity and stabilize π-π stacking interactions, making it suitable for applications in catalysis, sensing, and materials science. Its synthesis typically involves refluxing the free-base porphyrin with cobalt acetate in glacial acetic acid, a method analogous to other cobalt porphyrin complexes .
The compound exhibits unique electronic properties due to the electron-donating methyl groups, which influence redox potentials and ligand-binding affinities. Studies using quartz crystal microbalance (QCM) methods have demonstrated its adsorption behavior with metal ions like Al³⁺, Fe³⁺, and In³⁺, highlighting its utility in metalloporphyrin fabrication .
Preparation Methods
Synthetic Routes and Reaction Conditions
The synthesis of 5,10,15,20-Tetra(4-methylphenyl)-21H,23H-porphine cobalt typically involves the reaction of cobalt salts with the corresponding porphyrin ligand. One common method involves the reaction of cobalt(II) acetate with 5,10,15,20-Tetra(4-methylphenyl)porphyrin in a solvent such as toluene under reflux conditions . The reaction is monitored using spectroscopic techniques such as UV-Vis and IR spectroscopy to confirm the formation of the desired complex.
Industrial Production Methods
While specific industrial production methods for this compound are not extensively documented, the general approach would involve scaling up the laboratory synthesis procedures. This would include optimizing reaction conditions, using larger reaction vessels, and implementing purification techniques such as column chromatography or recrystallization to obtain the pure product.
Chemical Reactions Analysis
Redox Reactions and Electrochemical Behavior
The cobalt center in this porphyrin exhibits multiple accessible oxidation states, enabling participation in redox processes. Electrochemical studies of structurally analogous cobalt porphyrins reveal distinct redox potentials influenced by substituents:
Redox Process | E₁/₂ (V vs. SCE) | Peak Separation (ΔEₚ, mV) | Source |
---|---|---|---|
Coᴵᴵ → Coᴵ | -0.75 | 72 | |
Coᴵ → Co⁰ | -1.79 | 77 | |
Coᴵᴵᴵ → Coᴵᴵ | +0.29 | 379 |
These potentials indicate the compound’s ability to act as both an oxidizing and reducing agent. The fluorinated analog (3-fluorophenyl substituents) shows a positive shift in reduction potentials compared to non-fluorinated derivatives, suggesting electron-withdrawing groups enhance catalytic activity in CO₂ reduction .
Catalytic Oxidation Reactions
The complex demonstrates high efficiency in oxidation reactions due to its stable radical intermediates and axial ligand flexibility:
-
Alkane Oxidation : Activates C–H bonds in hydrocarbons via a proposed radical mechanism involving Coᴵᴵ/Coᴵᴵᴵ redox cycling.
-
Oxygen Evolution Reaction (OER) : Facilitates water oxidation under electrochemical conditions, with turnover frequencies (TOF) comparable to natural metalloenzymes.
CO₂ Electroreduction
While direct data for the 4-methylphenyl variant is limited, studies on similar cobalt porphyrins reveal:
-
Product Selectivity : Preferential formation of CO over formate (Faradaic efficiency >80% at -0.8 V vs. RHE) .
-
Mechanism : CO₂ binds to the Co⁰ state, followed by proton-coupled electron transfer to generate CO .
Axial Ligand Exchange Reactions
The cobalt center coordinates axial ligands (e.g., NO, CO, or pyridine), altering reactivity:
-
Nitric Oxide (NO) Binding : Forms Co–NO adducts, studied for applications in gas sensing and biomimetic catalysis .
-
Oxygen Binding : Reversible O₂ coordination mimics hemoglobin behavior, though stability depends on solvent and substituent electronic effects.
Comparative Reactivity with Analogous Complexes
Substituent effects significantly influence reactivity:
Mechanistic Insights
-
Electron Transfer : The porphyrin macrocycle stabilizes charge during redox transitions, as evidenced by UV-Vis spectral shifts (Soret band at 412 nm, Q-band at 532 nm) .
-
Ligand Effects : Electron-donating methyl groups enhance oxidative catalysis, while electron-withdrawing groups improve reductive processes .
This compound’s versatility in catalysis and redox chemistry makes it a valuable model for studying metalloporphyrin reactivity and designing advanced catalytic systems.
Scientific Research Applications
Catalytic Applications
1.1 CO2 Reduction
Cobalt porphyrins have been extensively studied for their potential in catalyzing the reduction of carbon dioxide (CO2). Research indicates that cobalt(II) porphyrins can effectively facilitate the electrochemical reduction of CO2 to valuable products such as carbon monoxide and formic acid. The efficiency of these reactions is influenced by the electronic properties of the porphyrin ligands and the nature of the cobalt center.
- Electrocatalytic Performance : Studies show that cobalt porphyrins exhibit varying electrocatalytic activity depending on their structural modifications. For instance, cobalt(II) 5,10,15,20-tetrakis(4-methylphenyl)porphyrin has demonstrated significant activity under alkaline conditions, with a reduction yield reaching approximately 0.68 µmol J^-1 when subjected to radiolytic reduction methods .
Catalyst Type | Product Yield (µmol J^-1) | Conditions |
---|---|---|
Cobalt(II) Porphyrin | 0.68 | Alkaline solution |
Cobalt Phthalocyanines | Varies | Carbon electrode deposition |
1.2 Dye Decolorization
Cobalt porphyrins have also been employed as catalysts for the decolorization of dyes in wastewater treatment processes. For example, cobalt(II) 5,10,15,20-tetrakis(4-methylphenyl)porphyrin has been shown to effectively decolorize methylene blue (MB) and crystal violet (CV) dyes in the presence of hydrogen peroxide. The mechanism involves the generation of reactive oxygen species that facilitate dye degradation.
- Dye Removal Efficiency : In comparative studies, cobalt porphyrins exhibited removal efficiencies of approximately 42% for CV and 44.2% for MB after a set reaction time .
Dye Type | Removal Efficiency (%) | Catalyst Used |
---|---|---|
Methylene Blue | 44.2 | Cobalt(II) Tetrakis(4-methylphenyl)porphyrin |
Crystal Violet | 42 | Cobalt(II) Tetrakis(4-methylphenyl)porphyrin |
Sensor Applications
Cobalt porphyrins are also recognized for their potential in sensor technology, particularly for detecting volatile organic compounds (VOCs). The unique electronic properties of cobalt porphyrins allow them to interact selectively with various small molecules.
- Colorimetric Sensor Arrays : Research has highlighted the ability of cobalt porphyrins to serve as effective colorimetric sensors for VOCs. The binding interactions lead to observable color changes that can be quantified for detection purposes .
Biological Applications
Cobalt porphyrins play crucial roles in biological systems due to their structural similarity to heme groups found in hemoglobin and other metalloproteins.
3.1 Oxygen Transport and Storage
Cobalt-containing porphyrins can mimic hemoglobin's function in oxygen transport and storage. Their ability to reversibly bind oxygen makes them candidates for developing artificial blood substitutes or oxygen carriers.
3.2 Antimicrobial Activity
Studies have indicated that certain cobalt porphyrins possess antimicrobial properties. Their effectiveness against various bacterial strains has been attributed to their ability to generate reactive oxygen species upon light activation .
Mechanism of Action
The mechanism by which 5,10,15,20-Tetra(4-methylphenyl)-21H,23H-porphine cobalt exerts its effects involves the coordination of the cobalt center to various substrates. This coordination can facilitate electron transfer processes, which are crucial in catalytic and photochemical reactions. The molecular targets and pathways involved depend on the specific application, such as the interaction with oxygen in oxidation reactions or the transfer of electrons in photochemical processes .
Comparison with Similar Compounds
Structural and Functional Comparison with Similar Compounds
Structural Modifications in Analogous Porphyrins
Key structural variations among cobalt porphyrins arise from substituents at the meso-phenyl positions. Below is a comparative analysis:
Key Observations :
- Electron-Donating Effects: Methoxy (CoTMeOPP) and amino (Co-TAPP) groups increase electron density at the porphyrin core compared to methyl groups in Co-TMPP²⁻, altering redox potentials and catalytic activity .
- Solubility: Pyridyl (H2TMPyP) and aminophenyl (Co-TAPP) substituents enhance aqueous solubility, whereas methyl and methoxy groups favor organic phases .
- Coordination Chemistry: Pyridyl and amino groups enable axial ligand binding or supramolecular assembly, whereas methyl groups prioritize non-covalent interactions (e.g., π-stacking) .
Insights :
- Electron-withdrawing groups (e.g., acetyloxy in H2TMAPP) improve yields due to stabilized intermediates .
- Methoxy-substituted porphyrins (CoTMeOPP) show lower yields, likely due to steric hindrance during metallation .
Electrochemical and Catalytic Properties
Redox potentials and catalytic performance are substituent-dependent:
Functional Differences :
- Co-TMPP²⁻’s methyl groups favor adsorption-driven applications (e.g., QCM sensors), whereas aminophenyl (Co-TAPP) and pyridyl (H2TMPyP) variants excel in electrocatalysis due to enhanced electron transfer .
- CoTMeOPP’s methoxy groups improve ORR activity but limit stability under acidic conditions compared to Co-TMPP²⁻ .
Biological Activity
Cobalt(2+);5,10,15,20-tetrakis(4-methylphenyl)porphyrin-22,23-diide (CoTMPP) is a cobalt-coordinated porphyrin complex that has garnered attention for its diverse biological activities, particularly in the fields of antimicrobial therapy and opioid neutralization. This article explores its mechanisms of action, efficacy in various biological contexts, and potential applications based on recent research findings.
1. Overview of Cobalt Porphyrins
Porphyrins are organic compounds that play crucial roles in biological systems, primarily due to their ability to coordinate metal ions. Cobalt porphyrins, such as CoTMPP, exhibit unique properties that make them suitable for various biomedical applications. The presence of cobalt enhances the reactivity of porphyrins towards biological targets.
2.1 Antimicrobial Activity
Cobalt porphyrins have been studied for their antimicrobial properties, particularly against antibiotic-resistant strains. A study demonstrated that cobalt-porphyrin complexes can generate reactive oxygen species (ROS) upon light activation, leading to significant bacterial cell damage. The mechanism involves:
- Photodynamic Therapy (PDT) : Upon irradiation with light, cobalt porphyrins can produce singlet oxygen (1O2), which damages cellular components such as membranes and nucleic acids.
- Cell Membrane Disruption : The amphipathic nature of porphyrins facilitates their accumulation in bacterial membranes, enhancing their phototoxic effects.
Table 1: Antimicrobial Efficacy of Cobalt Porphyrins
Bacterial Strain | MIC (µg/mL) | With Irradiation | Without Irradiation |
---|---|---|---|
MRSA | 69.42 | 54.71 | 109.30 |
P. aeruginosa | 54.71 | 402.90 | - |
S. aureus | 67.68 | 58.26 | - |
MIC = Minimum Inhibitory Concentration
2.2 Opioid Neutralization
Recent research highlights the ability of CoTMPP to neutralize synthetic opioids like fentanyl effectively. The cobalt complex has been shown to inhibit opioid receptor activation more effectively than other metal-porphyrin complexes.
- Mechanism : CoTMPP interacts with fentanyl to form breakdown adducts, leading to a reduction in its biological activity.
- Efficacy : In a tissue culture model, CoTMPP inhibited fentanyl activation of opioid receptors by approximately 42% compared to rhodium complexes.
Table 2: Efficacy of Cobalt Porphyrin in Opioid Neutralization
Complex Type | Percent Inhibition (%) |
---|---|
CoTMPP | 42 |
Rhodium Complex | Lower than CoTMPP |
3.1 Study on Antimicrobial Resistance
A study published in Photodynamic Antimicrobial Activity demonstrated the effectiveness of a similar cobalt porphyrin in combating MRSA and Pseudomonas infections under PDT conditions. The results indicated a significant reduction in bacterial viability when treated with cobalt porphyrins compared to controls.
3.2 Opioid Overdose Treatment
Research outlined in a patent application describes a method for utilizing CoTMPP as a therapeutic agent for opioid overdose by neutralizing the effects of synthetic opioids through its action on opioid receptors and enhancing the rate of fentanyl breakdown.
4. Conclusion
This compound exhibits promising biological activities that could be harnessed for therapeutic applications in antimicrobial treatment and opioid overdose management. Its mechanisms involve photodynamic effects and receptor inhibition, making it a versatile compound in modern medicine.
5. Future Directions
Further research is needed to explore:
- The cytotoxicity and genotoxicity profiles of cobalt porphyrins.
- Optimization of light activation parameters for enhanced antimicrobial efficacy.
- Clinical trials to validate the effectiveness of CoTMPP in treating opioid overdoses in humans.
Q & A
Basic Research Question: What are the optimal synthetic routes and characterization methods for cobalt(II) 5,10,15,20-tetrakis(4-methylphenyl)porphyrin?
Methodological Answer:
The cobalt(II) complex is synthesized via a two-step process:
Free-base porphyrin synthesis : Reflux pyrrole and 4-methylbenzaldehyde in propionic acid (138°C, 2 hours), followed by filtration and washing with deionized water. This yields 5,10,15,20-tetrakis(4-methylphenyl)porphyrin (H₂TTP) .
Metalation : Reflux H₂TTP with CoCl₂·2H₂O in pyridine (120°C, 6 hours) to form the cobalt(II) complex .
Characterization :
- UV-Vis spectroscopy identifies Soret and Q-bands to confirm metalation and electronic structure .
- IR spectroscopy detects shifts in N–H stretching (3448 cm⁻¹ in H₂TTP) and confirms metal coordination via pyrrole ring vibrations .
- Elemental analysis validates stoichiometry.
Basic Research Question: How does the central metal ion influence the photodynamic activity of tetrakis(4-methylphenyl)porphyrin complexes?
Methodological Answer:
Photodynamic activity is assessed via methylene blue degradation under visible light. Comparative studies show:
- Sn(II)H₂TTP exhibits higher activity than Co(II)H₂TTP , attributed to Sn(II)'s stronger Lewis acidity, enhancing singlet oxygen generation .
- H₂TTP (free base) shows minimal activity, emphasizing the necessity of metal coordination .
Experimental design : - Use controlled light intensity (e.g., 450 nm LED) and monitor degradation via UV-Vis kinetics.
- Compare quantum yields of singlet oxygen (¹O₂) using chemical traps like 1,3-diphenylisobenzofuran (DPBF) .
Advanced Research Question: How can substituent modifications on the porphyrin macrocycle enhance electrocatalytic oxygen reduction?
Methodological Answer:
Electrocatalytic performance is tuned by:
Peripheral substituents : Electron-withdrawing groups (e.g., –COOH, –F) stabilize intermediates during O₂ reduction. For example, Pt(II) moieties on cobalt porphyrins enhance π-backbonding, strengthening Co–O₂ interactions and increasing 4-electron pathway efficiency (~65% H₂O yield) .
Heteroatom substitution : Replacing pyrrole nitrogen with sulfur (e.g., dithiaporphyrins) alters redox potentials and improves conductivity .
Contradiction analysis : While electron-withdrawing groups enhance catalytic activity, they may reduce solubility. Balance via mixed substituents (e.g., –CH₃ for solubility and –COOH for catalysis) .
Advanced Research Question: What strategies resolve contradictions in reported photostability data for cobalt porphyrins?
Methodological Answer:
Discrepancies arise from:
- Light source variability : UV vs. visible light exposure (e.g., Sn(II)H₂TTP degrades faster under UV due to higher absorption ).
- Solvent effects : Polar solvents (e.g., DMF) accelerate degradation via radical pathways.
Resolution protocol : - Standardize light sources (e.g., AM1.5 solar simulator) and solvent systems.
- Use mass spectrometry to track decomposition products and identify degradation mechanisms .
Basic Research Question: What analytical applications exist for cobalt porphyrin derivatives in metal detection?
Methodological Answer:
Cobalt porphyrins act as ligands for heavy metal detection:
- HPLC analysis : Chelation with Cu²⁺ or Cd²⁺ shifts retention times, enabling simultaneous quantification (detection limit ~0.1 ppm) .
- UV-Vis spectroscopy : Metal coordination induces bathochromic shifts in Soret bands (e.g., Co²⁺ binding at 425 nm → 435 nm) .
Method optimization : Adjust pH (6–8) to stabilize metal–porphyrin complexes and minimize interference from competing ions .
Advanced Research Question: How do heteroatom substitutions in the porphyrin core affect electronic properties and reactivity?
Methodological Answer:
Substituting pyrrole nitrogens with sulfur or oxygen:
- Electronic effects : Sulfur lowers the LUMO energy, enhancing charge transfer (e.g., dithiaporphyrins show red-shifted Soret bands) .
- Reactivity : Thiaporphyrins exhibit stronger axial ligand binding, stabilizing Co(II)–peroxo intermediates in catalysis .
Experimental validation : - Compare cyclic voltammetry (CV) redox potentials of S₂TPP vs. H₂TPP.
- Use DFT calculations to model frontier molecular orbitals and predict reactivity .
Advanced Research Question: What methodologies optimize cobalt porphyrins for tumor diagnosis or photodynamic therapy (PDT)?
Methodological Answer:
Key design considerations:
Water solubility : Introduce –SO₃H or –COOH groups via post-synthetic modifications (e.g., sulfonation of 4-methylphenyl groups) .
Targeting : Conjugate with tumor-specific antibodies (e.g., anti-EGFR) using carbodiimide crosslinkers .
PDT efficiency : Measure ¹O₂ quantum yield under near-infrared (NIR) light for deeper tissue penetration .
Challenge : Balancing solubility with membrane permeability. Use liposomal encapsulation to enhance bioavailability .
Basic Research Question: What safety protocols are critical when handling cobalt porphyrins in laboratory settings?
Methodological Answer:
- Personal protective equipment (PPE) : Nitrile gloves, lab coats, and safety goggles to prevent skin/eye contact .
- Ventilation : Use fume hoods during synthesis to avoid inhaling pyridine or propionic acid vapors .
- Waste disposal : Collect organic residues in halogenated waste containers; neutralize acidic solutions before disposal .
Properties
Molecular Formula |
C48H36CoN4 |
---|---|
Molecular Weight |
727.8 g/mol |
IUPAC Name |
cobalt(2+);5,10,15,20-tetrakis(4-methylphenyl)porphyrin-22,23-diide |
InChI |
InChI=1S/C48H36N4.Co/c1-29-5-13-33(14-6-29)45-37-21-23-39(49-37)46(34-15-7-30(2)8-16-34)41-25-27-43(51-41)48(36-19-11-32(4)12-20-36)44-28-26-42(52-44)47(40-24-22-38(45)50-40)35-17-9-31(3)10-18-35;/h5-28H,1-4H3;/q-2;+2 |
InChI Key |
GAGCYGPTUZOPHZ-UHFFFAOYSA-N |
Canonical SMILES |
CC1=CC=C(C=C1)C2=C3C=CC(=C(C4=CC=C([N-]4)C(=C5C=CC(=N5)C(=C6C=CC2=N6)C7=CC=C(C=C7)C)C8=CC=C(C=C8)C)C9=CC=C(C=C9)C)[N-]3.[Co+2] |
Origin of Product |
United States |
Disclaimer and Information on In-Vitro Research Products
Please be aware that all articles and product information presented on BenchChem are intended solely for informational purposes. The products available for purchase on BenchChem are specifically designed for in-vitro studies, which are conducted outside of living organisms. In-vitro studies, derived from the Latin term "in glass," involve experiments performed in controlled laboratory settings using cells or tissues. It is important to note that these products are not categorized as medicines or drugs, and they have not received approval from the FDA for the prevention, treatment, or cure of any medical condition, ailment, or disease. We must emphasize that any form of bodily introduction of these products into humans or animals is strictly prohibited by law. It is essential to adhere to these guidelines to ensure compliance with legal and ethical standards in research and experimentation.