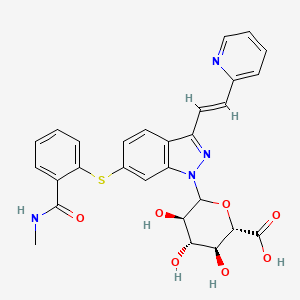
Axitinib N-Glucuronide
- Click on QUICK INQUIRY to receive a quote from our team of experts.
- With the quality product at a COMPETITIVE price, you can focus more on your research.
Overview
Description
Axitinib N-Glucuronide (M7) is a metabolite of axitinib , an orally active multikinase inhibitor . Axitinib itself is a potent and selective second-generation inhibitor of vascular endothelial growth factor receptors (VEGFR) 1, 2, and 3. It is approved for treating advanced renal cell carcinoma (RCC) after failure of one prior systemic therapy .
Chemical Reactions Analysis
Axitinib N-Glucuronide is a product of glucuronidation, where a glucuronic acid moiety is conjugated to axitinib. This process occurs primarily in the liver. The major metabolites in human plasma include M7 and axitinib sulfoxide (M12), both of which are considered pharmacologically inactive . Unfortunately, specific reagents and conditions for the glucuronidation reaction remain undisclosed.
Scientific Research Applications
Research on axitinib N-Glucuronide is limited, but its potential applications align with those of axitinib. These include:
Oncology: Investigating its role in cancer therapy, particularly in RCC.
Pharmacokinetics: Studying its metabolism and pharmacokinetic properties.
Drug Interactions: Assessing potential interactions with other drugs.
Mechanism of Action
Axitinib N-Glucuronide does not exert significant pharmacological effects itself. Instead, its parent compound, axitinib, inhibits VEGFRs, disrupting angiogenesis and tumor growth. The molecular targets and pathways involved are related to VEGF signaling.
Comparison with Similar Compounds
While M7 is unique due to its glucuronidation, other axitinib metabolites (such as M12) and related compounds (e.g., sorafenib, sunitinib) share similar mechanisms of action in targeting VEGFRs.
Biological Activity
Axitinib N-glucuronide is a significant metabolite of axitinib, a potent inhibitor of vascular endothelial growth factor receptors (VEGFRs). Understanding the biological activity of this compound is crucial for evaluating its pharmacological implications and potential therapeutic effects. This article delves into the metabolic pathways, enzymatic interactions, and biological efficacy of this compound, supported by relevant data tables and research findings.
Metabolic Pathways
Axitinib undergoes extensive metabolism primarily in the liver, where it is converted into various metabolites, including this compound. The primary enzyme responsible for this glucuronidation process is UDP-glucuronosyltransferase 1A1 (UGT1A1) , with minor contributions from other UGT isoforms such as UGT1A3, UGT1A4, and UGT1A9 .
Key Enzyme Kinetics
The kinetic parameters for the formation of this compound have been characterized as follows:
Parameter | Value |
---|---|
Km | 2.7 µM (HLM) |
Vmax | 8.9 pmol·min−1·mg−1 (HLM) |
Enzyme | UGT1A1 |
These values indicate that this compound formation is a relatively efficient process, highlighting the significant role of UGT1A1 in its metabolism .
Biological Activity and Pharmacodynamics
While axitinib itself exhibits potent inhibitory activity against VEGFRs, its metabolites, including this compound, demonstrate markedly reduced biological activity. In vitro studies have shown that this compound is approximately 8000-fold less potent against VEGFR-2 compared to axitinib . This diminished potency raises important considerations regarding the therapeutic efficacy and safety profile of axitinib in clinical settings.
Comparative Potency of Axitinib and Its Metabolites
Compound | IC50 (nM) |
---|---|
Axitinib | 0.06 - 0.3 |
This compound | >480 |
Axitinib Sulfoxide | >400 |
The data illustrates that while axitinib effectively inhibits VEGFR signaling pathways at low concentrations, its metabolites do not contribute significantly to this activity .
Case Studies and Clinical Implications
Several clinical studies have evaluated the pharmacokinetics of axitinib and its metabolites. For instance, a phase I trial demonstrated that the pharmacokinetic profile of axitinib is influenced by genetic polymorphisms affecting UGT enzymes and cytochrome P450 isoforms . Additionally, the presence of this compound in plasma was correlated with reduced therapeutic efficacy, emphasizing the importance of understanding metabolite dynamics in treatment outcomes.
Notable Observations
- Phase I Trials : These trials highlighted variability in patient responses based on genetic factors influencing drug metabolism.
- Adverse Effects : The reduced activity of this compound suggests that adverse effects may be more closely related to parent compound levels rather than its metabolites.
Properties
Molecular Formula |
C28H26N4O7S |
---|---|
Molecular Weight |
562.6 g/mol |
IUPAC Name |
(2S,3S,4S,5R)-3,4,5-trihydroxy-6-[6-[2-(methylcarbamoyl)phenyl]sulfanyl-3-[(E)-2-pyridin-2-ylethenyl]indazol-1-yl]oxane-2-carboxylic acid |
InChI |
InChI=1S/C28H26N4O7S/c1-29-26(36)18-7-2-3-8-21(18)40-16-10-11-17-19(12-9-15-6-4-5-13-30-15)31-32(20(17)14-16)27-24(35)22(33)23(34)25(39-27)28(37)38/h2-14,22-25,27,33-35H,1H3,(H,29,36)(H,37,38)/b12-9+/t22-,23-,24+,25-,27?/m0/s1 |
InChI Key |
YYKQABKSJYLETI-MRLOOFNMSA-N |
SMILES |
CNC(=O)C1=CC=CC=C1SC2=CC3=C(C=C2)C(=NN3C4C(C(C(C(O4)C(=O)O)O)O)O)C=CC5=CC=CC=N5 |
Isomeric SMILES |
CNC(=O)C1=CC=CC=C1SC2=CC3=C(C=C2)C(=NN3C4[C@@H]([C@H]([C@@H]([C@H](O4)C(=O)O)O)O)O)/C=C/C5=CC=CC=N5 |
Canonical SMILES |
CNC(=O)C1=CC=CC=C1SC2=CC3=C(C=C2)C(=NN3C4C(C(C(C(O4)C(=O)O)O)O)O)C=CC5=CC=CC=N5 |
Appearance |
Solid powder |
Purity |
>95% (or refer to the Certificate of Analysis) |
shelf_life |
>3 years if stored properly |
solubility |
Soluble in DMSO |
storage |
Dry, dark and at 0 - 4 C for short term (days to weeks) or -20 C for long term (months to years). |
Synonyms |
Axitinib N-Glucuronide |
Origin of Product |
United States |
Retrosynthesis Analysis
AI-Powered Synthesis Planning: Our tool employs the Template_relevance Pistachio, Template_relevance Bkms_metabolic, Template_relevance Pistachio_ringbreaker, Template_relevance Reaxys, Template_relevance Reaxys_biocatalysis model, leveraging a vast database of chemical reactions to predict feasible synthetic routes.
One-Step Synthesis Focus: Specifically designed for one-step synthesis, it provides concise and direct routes for your target compounds, streamlining the synthesis process.
Accurate Predictions: Utilizing the extensive PISTACHIO, BKMS_METABOLIC, PISTACHIO_RINGBREAKER, REAXYS, REAXYS_BIOCATALYSIS database, our tool offers high-accuracy predictions, reflecting the latest in chemical research and data.
Strategy Settings
Precursor scoring | Relevance Heuristic |
---|---|
Min. plausibility | 0.01 |
Model | Template_relevance |
Template Set | Pistachio/Bkms_metabolic/Pistachio_ringbreaker/Reaxys/Reaxys_biocatalysis |
Top-N result to add to graph | 6 |
Feasible Synthetic Routes
Disclaimer and Information on In-Vitro Research Products
Please be aware that all articles and product information presented on BenchChem are intended solely for informational purposes. The products available for purchase on BenchChem are specifically designed for in-vitro studies, which are conducted outside of living organisms. In-vitro studies, derived from the Latin term "in glass," involve experiments performed in controlled laboratory settings using cells or tissues. It is important to note that these products are not categorized as medicines or drugs, and they have not received approval from the FDA for the prevention, treatment, or cure of any medical condition, ailment, or disease. We must emphasize that any form of bodily introduction of these products into humans or animals is strictly prohibited by law. It is essential to adhere to these guidelines to ensure compliance with legal and ethical standards in research and experimentation.