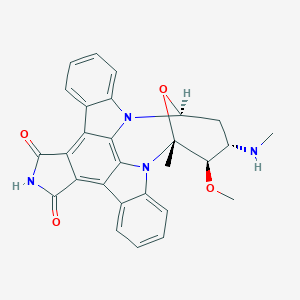
7-Oxostaurosporine
Overview
Description
7-oxo Staurosporine is a derivative of staurosporine, a natural product originally isolated from the bacterium Streptomyces staurosporeus. This compound belongs to the indolocarbazole family of alkaloids and is known for its diverse biological activities, including its potent inhibition of protein kinases.
Mechanism of Action
Target of Action
7-Oxostaurosporine is a potent inhibitor of protein kinase C (PKC), protein kinase A (PKA), phosphorylase kinase, EGFR, and c-Src . These proteins play crucial roles in cellular signaling, growth, and proliferation.
Mode of Action
This compound interacts with its targets by binding to the ATP-binding site on the kinase, thereby inhibiting their activity . This interaction leads to changes in cellular signaling pathways, affecting cell growth and proliferation.
Biochemical Pathways
The primary biochemical pathway affected by this compound is the protein kinase signaling pathway. By inhibiting key kinases, this compound disrupts the normal signaling processes within the cell, leading to changes in cell growth and proliferation .
Result of Action
This compound has been shown to induce cell cycle arrest in the G2/M phase in human leukemia K562 cells . This results in a decrease in cell proliferation and an increase in cell death. Additionally, this compound has been found to be cytotoxic to P388 mouse leukemia cells that are resistant and susceptible to doxorubicin .
Action Environment
The action of this compound can be influenced by various environmental factors. For instance, the compound was originally isolated from the bacterium S. platensis , suggesting that it may be more stable and effective in certain microbial environments.
Biochemical Analysis
Biochemical Properties
7-Oxostaurosporine is known to interact with various enzymes and proteins, particularly protein kinase C . It is a potent inhibitor of this enzyme, which plays a crucial role in several biochemical reactions, including cell proliferation and differentiation . The nature of these interactions involves the binding of this compound to the active site of the enzyme, thereby inhibiting its activity .
Cellular Effects
This compound has been found to exert significant effects on various types of cells. It influences cell function by impacting cell signaling pathways, gene expression, and cellular metabolism . For instance, it has been reported to induce cell cycle arrest in the G2/M phase in human leukemia K562 cells .
Molecular Mechanism
The molecular mechanism of action of this compound involves its binding interactions with biomolecules, enzyme inhibition or activation, and changes in gene expression . It inhibits the cell cycle at the G2 stage with the accumulation of 4C DNA cells .
Metabolic Pathways
Given its inhibitory effect on protein kinase C, it is likely that it interacts with enzymes and cofactors involved in signal transduction pathways .
Preparation Methods
Synthetic Routes and Reaction Conditions
The synthesis of 7-oxo Staurosporine involves several key steps, including the formation of the indolocarbazole core and subsequent functionalization. One common synthetic route starts with the electrophilic reaction of staurosporine derivatives with reagents such as N-chlorosuccinimide and N-bromosuccinimide to introduce halogen atoms at specific positions. This is followed by oxidation reactions using bases like potassium tert-butoxide to obtain the desired 7-oxo derivatives .
Industrial Production Methods
Industrial production of 7-oxo Staurosporine can be achieved through heterologous expression and process optimization. By integrating multi-copy biosynthetic gene clusters into well-characterized heterologous hosts such as Streptomyces albus, and optimizing fermentation processes, high yields of 7-oxo Staurosporine can be obtained. This method has been shown to significantly increase production levels compared to native producers .
Chemical Reactions Analysis
Types of Reactions
7-oxo Staurosporine undergoes various chemical reactions, including oxidation, reduction, and substitution. For example, oxidation reactions can be used to introduce oxygen-containing functional groups, while substitution reactions can modify the compound’s structure to enhance its biological activity .
Common Reagents and Conditions
Common reagents used in the synthesis and modification of 7-oxo Staurosporine include N-chlorosuccinimide, N-bromosuccinimide, and potassium tert-butoxide.
Major Products Formed
The major products formed from these reactions include various halogenated and oxidized derivatives of 7-oxo Staurosporine. These derivatives have been shown to exhibit enhanced biological activities, making them valuable for further research and development .
Scientific Research Applications
Comparison with Similar Compounds
Similar Compounds
Staurosporine: The parent compound, known for its broad-spectrum kinase inhibition but limited clinical use due to its lack of selectivity.
7-hydroxystaurosporine (UCN-01): A derivative with enhanced selectivity for certain kinases, currently in clinical trials for cancer therapy.
Midostaurin: A semi-synthetic derivative used for the treatment of acute myeloid leukemia.
Uniqueness of 7-oxo Staurosporine
7-oxo Staurosporine is unique due to its specific modifications at the 7-position, which enhance its biological activity and selectivity compared to the parent compound. These modifications make it a promising candidate for further development as an anticancer agent .
Properties
IUPAC Name |
(2S,3R,4R,6R)-3-methoxy-2-methyl-4-(methylamino)-29-oxa-1,7,17-triazaoctacyclo[12.12.2.12,6.07,28.08,13.015,19.020,27.021,26]nonacosa-8,10,12,14,19,21,23,25,27-nonaene-16,18-dione | |
---|---|---|
Source | PubChem | |
URL | https://pubchem.ncbi.nlm.nih.gov | |
Description | Data deposited in or computed by PubChem | |
InChI |
InChI=1S/C28H24N4O4/c1-28-25(35-3)15(29-2)12-18(36-28)31-16-10-6-4-8-13(16)19-21-22(27(34)30-26(21)33)20-14-9-5-7-11-17(14)32(28)24(20)23(19)31/h4-11,15,18,25,29H,12H2,1-3H3,(H,30,33,34)/t15-,18-,25-,28+/m1/s1 | |
Source | PubChem | |
URL | https://pubchem.ncbi.nlm.nih.gov | |
Description | Data deposited in or computed by PubChem | |
InChI Key |
POTTVLREWUNNRO-UGZRAAABSA-N | |
Source | PubChem | |
URL | https://pubchem.ncbi.nlm.nih.gov | |
Description | Data deposited in or computed by PubChem | |
Canonical SMILES |
CC12C(C(CC(O1)N3C4=CC=CC=C4C5=C6C(=C7C8=CC=CC=C8N2C7=C53)C(=O)NC6=O)NC)OC | |
Source | PubChem | |
URL | https://pubchem.ncbi.nlm.nih.gov | |
Description | Data deposited in or computed by PubChem | |
Isomeric SMILES |
C[C@@]12[C@@H]([C@@H](C[C@@H](O1)N3C4=CC=CC=C4C5=C6C(=C7C8=CC=CC=C8N2C7=C53)C(=O)NC6=O)NC)OC | |
Source | PubChem | |
URL | https://pubchem.ncbi.nlm.nih.gov | |
Description | Data deposited in or computed by PubChem | |
Molecular Formula |
C28H24N4O4 | |
Source | PubChem | |
URL | https://pubchem.ncbi.nlm.nih.gov | |
Description | Data deposited in or computed by PubChem | |
Molecular Weight |
480.5 g/mol | |
Source | PubChem | |
URL | https://pubchem.ncbi.nlm.nih.gov | |
Description | Data deposited in or computed by PubChem | |
Retrosynthesis Analysis
AI-Powered Synthesis Planning: Our tool employs the Template_relevance Pistachio, Template_relevance Bkms_metabolic, Template_relevance Pistachio_ringbreaker, Template_relevance Reaxys, Template_relevance Reaxys_biocatalysis model, leveraging a vast database of chemical reactions to predict feasible synthetic routes.
One-Step Synthesis Focus: Specifically designed for one-step synthesis, it provides concise and direct routes for your target compounds, streamlining the synthesis process.
Accurate Predictions: Utilizing the extensive PISTACHIO, BKMS_METABOLIC, PISTACHIO_RINGBREAKER, REAXYS, REAXYS_BIOCATALYSIS database, our tool offers high-accuracy predictions, reflecting the latest in chemical research and data.
Strategy Settings
Precursor scoring | Relevance Heuristic |
---|---|
Min. plausibility | 0.01 |
Model | Template_relevance |
Template Set | Pistachio/Bkms_metabolic/Pistachio_ringbreaker/Reaxys/Reaxys_biocatalysis |
Top-N result to add to graph | 6 |
Feasible Synthetic Routes
Disclaimer and Information on In-Vitro Research Products
Please be aware that all articles and product information presented on BenchChem are intended solely for informational purposes. The products available for purchase on BenchChem are specifically designed for in-vitro studies, which are conducted outside of living organisms. In-vitro studies, derived from the Latin term "in glass," involve experiments performed in controlled laboratory settings using cells or tissues. It is important to note that these products are not categorized as medicines or drugs, and they have not received approval from the FDA for the prevention, treatment, or cure of any medical condition, ailment, or disease. We must emphasize that any form of bodily introduction of these products into humans or animals is strictly prohibited by law. It is essential to adhere to these guidelines to ensure compliance with legal and ethical standards in research and experimentation.