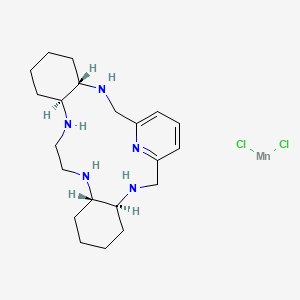
Avasopasem manganese
- Click on QUICK INQUIRY to receive a quote from our team of experts.
- With the quality product at a COMPETITIVE price, you can focus more on your research.
Overview
Description
Avasopasem manganese is a superoxide dismutase mimetic drug candidate.
Scientific Research Applications
Manganese-Enhanced MRI of the Brain
Avasopasem manganese, in the form of mangafodipir, has been used as a contrast agent for manganese-enhanced MRI (Magnetic Resonance Imaging) of the brain and glandular structures in the rostral head and neck. The research demonstrates that manganese ions from mangafodipir enable noninvasive visualization of structures outside the blood-brain barrier without adverse clinical effects, paving the way for future neuroradiological investigations in diseases (Sudarshana et al., 2019).
Manganese Homeostasis and Disease
A cohort of patients with a novel autosomal recessive manganese transporter defect caused by mutations in SLC39A14 has been identified. This defect leads to excessive accumulation of manganese, resulting in rapidly progressive childhood-onset parkinsonism-dystonia. The study highlights that SLC39A14 functions as a pivotal manganese transporter in vertebrates and suggests potential therapeutic interventions, such as chelation, to manage this condition (Tuschl et al., 2016).
Manganese in Environmental Health
Excessive exposure to manganese has been associated with intellectual impairment in children. Manganese in drinking water, especially at levels common in groundwater, has been linked to lower IQ scores in children, suggesting that manganese exposure can be a public health concern (Bouchard et al., 2010). Moreover, environmental exposure to manganese through air or water has been implicated in various neurobehavioral effects, from neurodevelopment in infants to neurocognitive deficits in adults, highlighting the need for monitoring and potential interventions in affected communities (Hernández-Bonilla et al., 2016).
properties
CAS RN |
435327-40-5 |
---|---|
Molecular Formula |
C21H35Cl2MnN5 |
Molecular Weight |
483.38 |
IUPAC Name |
dichloromanganese;(4S,9S,14S,19S)-3,10,13,20,26-pentazatetracyclo[20.3.1.04,9.014,19]hexacosa-1(26),22,24-triene |
InChI |
InChI=1S/C21H35N5.2ClH.Mn/c1-3-10-20-18(8-1)22-12-13-23-19-9-2-4-11-21(19)25-15-17-7-5-6-16(26-17)14-24-20;;;/h5-7,18-25H,1-4,8-15H2;2*1H;/q;;;+2/p-2/t18-,19-,20-,21-;;;/m0.../s1 |
InChI Key |
WXEMWBBXVXHEPU-NDOCQCNASA-L |
SMILES |
C1CCC2C(C1)NCCNC3CCCCC3NCC4=CC=CC(=N4)CN2.Cl[Mn]Cl |
Appearance |
Solid powder |
Purity |
>98% (or refer to the Certificate of Analysis) |
shelf_life |
>3 years if stored properly |
solubility |
Soluble in DMSO |
storage |
Dry, dark and at 0 - 4 C for short term (days to weeks) or -20 C for long term (months to years). |
synonyms |
Avasopasem manganese; |
Origin of Product |
United States |
Retrosynthesis Analysis
AI-Powered Synthesis Planning: Our tool employs the Template_relevance Pistachio, Template_relevance Bkms_metabolic, Template_relevance Pistachio_ringbreaker, Template_relevance Reaxys, Template_relevance Reaxys_biocatalysis model, leveraging a vast database of chemical reactions to predict feasible synthetic routes.
One-Step Synthesis Focus: Specifically designed for one-step synthesis, it provides concise and direct routes for your target compounds, streamlining the synthesis process.
Accurate Predictions: Utilizing the extensive PISTACHIO, BKMS_METABOLIC, PISTACHIO_RINGBREAKER, REAXYS, REAXYS_BIOCATALYSIS database, our tool offers high-accuracy predictions, reflecting the latest in chemical research and data.
Strategy Settings
Precursor scoring | Relevance Heuristic |
---|---|
Min. plausibility | 0.01 |
Model | Template_relevance |
Template Set | Pistachio/Bkms_metabolic/Pistachio_ringbreaker/Reaxys/Reaxys_biocatalysis |
Top-N result to add to graph | 6 |
Feasible Synthetic Routes
Disclaimer and Information on In-Vitro Research Products
Please be aware that all articles and product information presented on BenchChem are intended solely for informational purposes. The products available for purchase on BenchChem are specifically designed for in-vitro studies, which are conducted outside of living organisms. In-vitro studies, derived from the Latin term "in glass," involve experiments performed in controlled laboratory settings using cells or tissues. It is important to note that these products are not categorized as medicines or drugs, and they have not received approval from the FDA for the prevention, treatment, or cure of any medical condition, ailment, or disease. We must emphasize that any form of bodily introduction of these products into humans or animals is strictly prohibited by law. It is essential to adhere to these guidelines to ensure compliance with legal and ethical standards in research and experimentation.