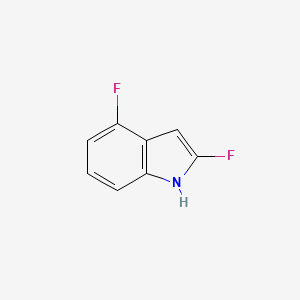
2,4-difluoro-1H-indole
- Click on QUICK INQUIRY to receive a quote from our team of experts.
- With the quality product at a COMPETITIVE price, you can focus more on your research.
Overview
Description
2,4-Difluoro-1H-indole is a fluorinated derivative of indole, a significant heterocyclic compound. Indole derivatives are known for their diverse biological activities and applications in various fields, including pharmaceuticals, agriculture, and materials science
Preparation Methods
Synthetic Routes and Reaction Conditions: One common method is the electrophilic fluorination of 1H-indole using fluorinating agents such as Selectfluor or N-fluorobenzenesulfonimide (NFSI) under mild conditions . Another approach involves the use of palladium-catalyzed cross-coupling reactions, such as the Suzuki-Miyaura coupling, to introduce fluorinated substituents onto the indole ring .
Industrial Production Methods: Industrial production of 2,4-difluoro-1H-indole may involve large-scale fluorination processes using continuous flow reactors to ensure efficient and controlled fluorination. The use of environmentally benign fluorinating agents and catalysts is also emphasized to minimize the environmental impact .
Chemical Reactions Analysis
Types of Reactions: 2,4-Difluoro-1H-indole can undergo various chemical reactions, including:
Electrophilic Substitution:
Nucleophilic Substitution: The presence of fluorine atoms can activate the indole ring towards nucleophilic substitution reactions, leading to the formation of diverse derivatives.
Oxidation and Reduction: The compound can undergo oxidation and reduction reactions to form different oxidation states and derivatives.
Common Reagents and Conditions:
Electrophilic Substitution: Reagents such as halogens, nitrating agents, and sulfonating agents under acidic conditions.
Nucleophilic Substitution: Reagents such as amines, thiols, and alkoxides under basic conditions.
Oxidation: Reagents such as potassium permanganate or chromium trioxide.
Reduction: Reagents such as lithium aluminum hydride or sodium borohydride.
Major Products Formed: The major products formed from these reactions include various substituted indoles, which can be further functionalized for specific applications .
Scientific Research Applications
2,4-Difluoro-1H-indole has a wide range of applications in scientific research:
Mechanism of Action
The mechanism of action of 2,4-difluoro-1H-indole involves its interaction with specific molecular targets and pathways. The fluorine atoms can enhance the compound’s binding affinity to biological targets, such as enzymes and receptors, by forming strong hydrogen bonds and hydrophobic interactions . This can lead to the modulation of various biological pathways, resulting in the desired therapeutic effects .
Comparison with Similar Compounds
- 2-Fluoro-1H-indole
- 4-Fluoro-1H-indole
- 2,4-Dichloro-1H-indole
Comparison: 2,4-Difluoro-1H-indole is unique due to the presence of two fluorine atoms at specific positions on the indole ring, which can significantly influence its chemical and biological properties. Compared to its mono-fluorinated counterparts, this compound may exhibit enhanced stability, increased lipophilicity, and improved binding affinity to biological targets .
Properties
Molecular Formula |
C8H5F2N |
---|---|
Molecular Weight |
153.13 g/mol |
IUPAC Name |
2,4-difluoro-1H-indole |
InChI |
InChI=1S/C8H5F2N/c9-6-2-1-3-7-5(6)4-8(10)11-7/h1-4,11H |
InChI Key |
JGYMIVIOMGCKTM-UHFFFAOYSA-N |
Canonical SMILES |
C1=CC2=C(C=C(N2)F)C(=C1)F |
Origin of Product |
United States |
Disclaimer and Information on In-Vitro Research Products
Please be aware that all articles and product information presented on BenchChem are intended solely for informational purposes. The products available for purchase on BenchChem are specifically designed for in-vitro studies, which are conducted outside of living organisms. In-vitro studies, derived from the Latin term "in glass," involve experiments performed in controlled laboratory settings using cells or tissues. It is important to note that these products are not categorized as medicines or drugs, and they have not received approval from the FDA for the prevention, treatment, or cure of any medical condition, ailment, or disease. We must emphasize that any form of bodily introduction of these products into humans or animals is strictly prohibited by law. It is essential to adhere to these guidelines to ensure compliance with legal and ethical standards in research and experimentation.