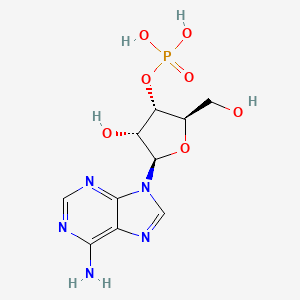
3'-Adenylic acid
Overview
Description
3’-Adenylic acid, also known as adenosine 3’-monophosphate, is a nucleotide that plays a crucial role in various biological processes. It consists of a phosphate group, the sugar ribose, and the nucleobase adenine. This compound is an ester of phosphoric acid and the nucleoside adenosine .
Mechanism of Action
Target of Action
3’-Adenylic acid, also known as Adenosine Monophosphate (AMP), is a nucleotide that plays a crucial role in many cellular metabolic processes . It primarily targets enzymes such as myophosphorylase-b, which it allosterically activates .
Mode of Action
AMP is an ester of phosphoric acid with the nucleoside adenosine . It consists of a phosphate group, the pentose sugar ribose, and the nucleobase adenine . It affects a number of immune functions, including the reversal of malnutrition and starvation-induced immunosuppression, the enhancement of T-cell maturation and function, the enhancement of natural killer cell activity, the improvement of delayed cutaneous hypersensitivity, and the modulation of T-cell responses toward type 1 CD4 helper lymphocytes or Th1 cells .
Biochemical Pathways
AMP plays a significant role in various biochemical pathways. It is interconverted to Adenosine Triphosphate (ATP) and Adenosine Diphosphate (ADP) in many cellular metabolic processes . When RNA is broken down by living systems, nucleoside monophosphates, including adenosine monophosphate, are formed .
Pharmacokinetics
It is known that amp can be produced from adp by the myokinase (adenylate kinase) reaction when the atp reservoir in the cell is low . AMP can also be formed by hydrolysis of ATP into AMP and pyrophosphate .
Result of Action
The action of AMP results in various molecular and cellular effects. It is used as a dietary supplement to boost immune activity, and is also used as a substitute sweetener to aid in the maintenance of a low-calorie diet . It also plays a role in the synthesis of RNA .
Biochemical Analysis
Biochemical Properties
3’-Adenylic acid is integral to many biochemical reactions. It is interconverted to adenosine triphosphate (ATP) and adenosine diphosphate (ADP), which are essential for energy transfer within cells . The compound interacts with enzymes such as adenylate kinase, which catalyzes the conversion of two molecules of ADP into ATP and AMP. Additionally, 3’-Adenylic acid is involved in the activation of enzymes like myophosphorylase-b, which plays a role in glycogen metabolism .
Cellular Effects
3’-Adenylic acid influences various cellular processes, including cell signaling pathways, gene expression, and cellular metabolism. It acts as a signaling molecule that can activate protein kinases, leading to the phosphorylation of target proteins and subsequent changes in cellular activities . The compound also affects gene expression by serving as a precursor for cyclic adenosine monophosphate (cAMP), a secondary messenger that regulates transcription factors and other proteins involved in gene regulation .
Molecular Mechanism
At the molecular level, 3’-Adenylic acid exerts its effects through binding interactions with various biomolecules. It can bind to and activate adenylate cyclase, an enzyme that converts ATP to cAMP . This activation leads to a cascade of intracellular events, including the activation of protein kinase A (PKA), which phosphorylates multiple target proteins, thereby modulating their activity . Additionally, 3’-Adenylic acid can be converted into inosine monophosphate by the enzyme myoadenylate deaminase, which plays a role in purine metabolism .
Temporal Effects in Laboratory Settings
In laboratory settings, the effects of 3’-Adenylic acid can change over time due to its stability and degradation. The compound is relatively stable under physiological conditions but can be hydrolyzed by phosphodiesterases, leading to the formation of adenosine and inorganic phosphate . Long-term studies have shown that 3’-Adenylic acid can influence cellular functions such as energy metabolism and gene expression over extended periods .
Dosage Effects in Animal Models
The effects of 3’-Adenylic acid vary with different dosages in animal models. At low doses, it can enhance cellular energy levels and improve metabolic functions . At high doses, it may lead to toxic effects, including disruptions in cellular signaling pathways and metabolic imbalances . Threshold effects have been observed, where a certain dosage is required to elicit a significant biological response .
Metabolic Pathways
3’-Adenylic acid is involved in several metabolic pathways, including the purine nucleotide cycle and glycolysis . It can be converted into inosine monophosphate by myoadenylate deaminase, which is then further metabolized to uric acid for excretion . The compound also plays a role in the regulation of glycolytic enzymes, influencing metabolic flux and the levels of various metabolites .
Transport and Distribution
Within cells, 3’-Adenylic acid is transported and distributed by specific transporters and binding proteins. It is taken up by cells through nucleoside transporters and can be localized to various cellular compartments, including the cytoplasm and mitochondria . The distribution of 3’-Adenylic acid within tissues is influenced by its interactions with binding proteins that facilitate its transport and accumulation .
Subcellular Localization
3’-Adenylic acid is localized to specific subcellular compartments, where it exerts its biological functions. It is found in the cytoplasm, where it participates in energy metabolism and signaling pathways . Additionally, it can be targeted to the mitochondria, where it plays a role in oxidative phosphorylation and ATP synthesis . The localization of 3’-Adenylic acid is regulated by targeting signals and post-translational modifications that direct it to specific organelles .
Preparation Methods
Synthetic Routes and Reaction Conditions: 3’-Adenylic acid can be synthesized through the phosphorylation of adenosine. The reaction typically involves the use of phosphorylating agents such as phosphorus oxychloride (POCl3) in the presence of a base like pyridine. The reaction conditions often require anhydrous solvents and controlled temperatures to ensure the selective formation of the 3’-phosphate ester .
Industrial Production Methods: In industrial settings, 3’-Adenylic acid is often produced through enzymatic methods. Enzymes such as adenosine kinase can catalyze the phosphorylation of adenosine to form 3’-Adenylic acid. This method is preferred due to its specificity and efficiency .
Types of Reactions:
Oxidation: 3’-Adenylic acid can undergo oxidation reactions, particularly at the adenine moiety. Common oxidizing agents include hydrogen peroxide and potassium permanganate.
Reduction: Reduction reactions are less common but can occur under specific conditions using reducing agents like sodium borohydride.
Common Reagents and Conditions:
Oxidation: Hydrogen peroxide, potassium permanganate.
Reduction: Sodium borohydride.
Substitution: Various nucleophiles under acidic or basic conditions.
Major Products:
Oxidation: Oxidized derivatives of adenine.
Reduction: Reduced forms of the ribose or adenine moiety.
Substitution: Nucleotide analogs with modified phosphate groups.
Scientific Research Applications
3’-Adenylic acid has a wide range of applications in scientific research:
Chemistry: It is used as a precursor in the synthesis of other nucleotides and nucleotide analogs.
Biology: It plays a role in cellular signaling pathways and is involved in the regulation of various metabolic processes.
Medicine: 3’-Adenylic acid is studied for its potential therapeutic applications, including its role in immune modulation and as a potential treatment for certain metabolic disorders.
Industry: It is used in the production of nucleotide-based products, such as dietary supplements and pharmaceuticals
Comparison with Similar Compounds
Adenosine 5’-monophosphate: Similar in structure but with the phosphate group attached at the 5’ position.
Inosine monophosphate: Contains hypoxanthine instead of adenine.
Guanosine monophosphate: Contains guanine instead of adenine.
Uniqueness: 3’-Adenylic acid is unique due to the specific positioning of the phosphate group at the 3’ position, which influences its biochemical properties and interactions. This distinct structure allows it to participate in specific enzymatic reactions and signaling pathways that are not accessible to its analogs .
Properties
IUPAC Name |
[5-(6-aminopurin-9-yl)-4-hydroxy-2-(hydroxymethyl)oxolan-3-yl] dihydrogen phosphate | |
---|---|---|
Details | Computed by Lexichem TK 2.7.0 (PubChem release 2021.05.07) | |
Source | PubChem | |
URL | https://pubchem.ncbi.nlm.nih.gov | |
Description | Data deposited in or computed by PubChem | |
InChI |
InChI=1S/C10H14N5O7P/c11-8-5-9(13-2-12-8)15(3-14-5)10-6(17)7(4(1-16)21-10)22-23(18,19)20/h2-4,6-7,10,16-17H,1H2,(H2,11,12,13)(H2,18,19,20) | |
Details | Computed by InChI 1.0.6 (PubChem release 2021.05.07) | |
Source | PubChem | |
URL | https://pubchem.ncbi.nlm.nih.gov | |
Description | Data deposited in or computed by PubChem | |
InChI Key |
LNQVTSROQXJCDD-UHFFFAOYSA-N | |
Details | Computed by InChI 1.0.6 (PubChem release 2021.05.07) | |
Source | PubChem | |
URL | https://pubchem.ncbi.nlm.nih.gov | |
Description | Data deposited in or computed by PubChem | |
Canonical SMILES |
C1=NC(=C2C(=N1)N(C=N2)C3C(C(C(O3)CO)OP(=O)(O)O)O)N | |
Details | Computed by OEChem 2.3.0 (PubChem release 2021.05.07) | |
Source | PubChem | |
URL | https://pubchem.ncbi.nlm.nih.gov | |
Description | Data deposited in or computed by PubChem | |
Molecular Formula |
C10H14N5O7P | |
Details | Computed by PubChem 2.1 (PubChem release 2021.05.07) | |
Source | PubChem | |
URL | https://pubchem.ncbi.nlm.nih.gov | |
Description | Data deposited in or computed by PubChem | |
Molecular Weight |
347.22 g/mol | |
Details | Computed by PubChem 2.1 (PubChem release 2021.05.07) | |
Source | PubChem | |
URL | https://pubchem.ncbi.nlm.nih.gov | |
Description | Data deposited in or computed by PubChem | |
CAS No. |
84-21-9 | |
Record name | 3'-Adenylic acid | |
Source | DTP/NCI | |
URL | https://dtp.cancer.gov/dtpstandard/servlet/dwindex?searchtype=NSC&outputformat=html&searchlist=210570 | |
Description | The NCI Development Therapeutics Program (DTP) provides services and resources to the academic and private-sector research communities worldwide to facilitate the discovery and development of new cancer therapeutic agents. | |
Explanation | Unless otherwise indicated, all text within NCI products is free of copyright and may be reused without our permission. Credit the National Cancer Institute as the source. | |
Q1: What are the main biological effects of 3'-adenylic acid on cell division and the mitotic cycle?
A1: Research suggests that this compound exhibits an inhibitory effect on cell division. [] This inhibition is linked to a significant increase in the duration of the mitotic cycle, as observed in Vicia faba. [] It's proposed that the compound's strong influence on the nucleus and chromosomes, particularly the induction of picnosis, stems from its potential to cause DNA depolymerization. [] This disruption of DNA integrity could further impede the synthesis of macromolecules. []
Q2: What are the key differences in this compound content observed in various marine organisms, and what enzymatic basis explains these variations?
A2: Significant variations in this compound levels are observed across different marine species. For instance, salted clam and cuttlefish pickles exhibit high this compound concentrations compared to other seafood pickles. [] This difference is attributed to the absence of adenylic deaminase in clams and certain invertebrates, leading to the accumulation of adenylic acid and classifying them as AMP-type organisms. [] In contrast, salted yellow tail pickles contain lower levels of this compound but significant amounts of 5'-inosinic acid, characterizing them as IMP-type. [] This difference highlights the diverse metabolic pathways and enzyme activities present in various marine organisms.
Q3: How does the presence of this compound contribute to the distinct flavor profiles of certain foods?
A3: this compound, along with other nucleotides and amino acids, plays a crucial role in shaping the unique flavor profiles of various foods. For example, the characteristic flavor of salted oyster pickles is attributed to a high concentration of this compound and the presence of sweet amino acids like lysine, threonine, isoleucine, and leucine. [] Additionally, the combined effect of this compound with glutamic acid and aspartic acid contributes to the specific taste of salted yellow tail pickles, demonstrating a potential synergistic interaction between these flavor components. []
Q4: Can you elaborate on the metabolic pathways affected by sodium fluoride (NaF) stress in silkworms, particularly those involving this compound?
A4: Metabolomic analysis of silkworm midgut tissue exposed to NaF stress revealed significant alterations in purine metabolism, particularly in fluoride-tolerant strains. [] A notable observation was the upregulation of this compound and hypoxanthine, alongside the downregulation of guanine, allantoic acid, xanthine, N-acetyl-L-glutamic acid, and pyruvate. [] These findings suggest a potential link between these metabolic pathways, including those involving this compound, and the mechanisms underlying NaF tolerance in silkworms.
Q5: What is the role of microbial ribonucleases in the context of this compound?
A5: Microbial ribonucleases, specifically those exhibiting this compound specificity, like RNase T2 from Aspergillus oryzae, play a crucial role in RNA degradation by cleaving phosphodiester bonds adjacent to this compound residues. [, ] This degradation process is essential for generating mature, functional RNA molecules from their precursors. [] The discovery and characterization of these enzymes highlight the importance of microbial sources in understanding RNA metabolism and the diverse roles of ribonucleases in biological systems.
Disclaimer and Information on In-Vitro Research Products
Please be aware that all articles and product information presented on BenchChem are intended solely for informational purposes. The products available for purchase on BenchChem are specifically designed for in-vitro studies, which are conducted outside of living organisms. In-vitro studies, derived from the Latin term "in glass," involve experiments performed in controlled laboratory settings using cells or tissues. It is important to note that these products are not categorized as medicines or drugs, and they have not received approval from the FDA for the prevention, treatment, or cure of any medical condition, ailment, or disease. We must emphasize that any form of bodily introduction of these products into humans or animals is strictly prohibited by law. It is essential to adhere to these guidelines to ensure compliance with legal and ethical standards in research and experimentation.