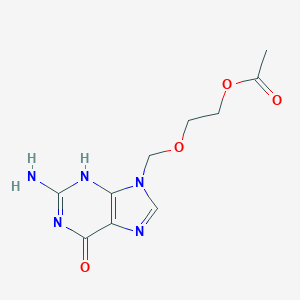
Acyclovir Acetate
Overview
Description
Acyclovir Acetate is a synthetic compound with the molecular formula C10H13N5O4. It is a derivative of guanine, a nucleobase found in DNA and RNA. This compound is of significant interest due to its potential antiviral properties and its role as an intermediate in the synthesis of antiviral drugs such as acyclovir.
Mechanism of Action
Acyclovir Acetate, also known as “9-(2-Acetoxyethoxymethyl)guanine” or “9-(2’-ACETOXYETHOXYMETHYL)GUANINE”, is a synthetic acyclic nucleoside analogue . It is primarily used for the treatment of herpes simplex virus infections, chickenpox, and shingles .
Target of Action
This compound primarily targets herpes simplex virus types 1 and 2 (HSV-1, HSV-2) and varicella-zoster virus (VZV) . These viruses are responsible for conditions such as herpes simplex, varicella zoster, and herpes zoster .
Mode of Action
This compound works by selectively inhibiting the replication of the herpes simplex virus types 1 and 2 (HSV-1, HSV-2) and varicella-zoster virus (VZV) . After intracellular uptake, it is converted to acyclovir monophosphate by virally-encoded thymidine kinase .
Biochemical Pathways
The monophosphate derivative of this compound is subsequently converted to acyclovir triphosphate by cellular enzymes . Acyclovir triphosphate competitively inhibits viral DNA polymerase by acting as an analog to deoxyguanosine triphosphate (dGTP) . Incorporation of acyclovir triphosphate into DNA results in chain termination since the absence of a 3’ hydroxyl group prevents the attachment of additional nucleosides .
Pharmacokinetics
The bioavailability of this compound after oral administration is approximately 15–20% . It is metabolized in the liver and has an elimination half-life of 2–4 hours . The majority of the drug (62–90%) is excreted unchanged in the urine .
Result of Action
The result of this compound’s action is the decreased production of the virus’s DNA . This inhibits the replication of the virus, thereby treating the infection . It is generally used first line in the treatment of these viruses .
Action Environment
The action of this compound can be influenced by various environmental factors. For instance, the presence of viral thymidine kinase is crucial for the conversion of this compound to its monophosphate form . Additionally, the drug’s efficacy can be affected by the patient’s immune status, with immunocompromised patients potentially requiring higher doses or longer treatment durations .
Biochemical Analysis
Biochemical Properties
Acyclovir Acetate interacts with various enzymes, proteins, and other biomolecules. It is active against herpes simplex virus type 1 and 2 (HSV-1 and HSV-2) . The central composite design (CCD) of Design Expert (quality by design tool) was employed for identification of significant attributes (flow rate and concentration of buffer), which affected the performance of the developed method .
Cellular Effects
The effects of this compound on various types of cells and cellular processes are significant. It influences cell function, including any impact on cell signaling pathways, gene expression, and cellular metabolism . The toxic effect exerted by this compound on CEM and Vero cells in culture limits the utility of this compound as an antiviral agent .
Molecular Mechanism
This compound exerts its effects at the molecular level, including any binding interactions with biomolecules, enzyme inhibition or activation, and changes in gene expression . The mechanism driving this compound’s ability to inhibit viral coding, starting from its development and pharmacology, is a subject of extensive research .
Temporal Effects in Laboratory Settings
The changes in the effects of this compound over time in laboratory settings include information on the product’s stability, degradation, and any long-term effects on cellular function observed in in vitro or in vivo studies .
Dosage Effects in Animal Models
The effects of this compound vary with different dosages in animal models. This includes any threshold effects observed in these studies, as well as any toxic or adverse effects at high doses .
Metabolic Pathways
This compound is involved in various metabolic pathways, including any enzymes or cofactors that it interacts with. This also includes any effects on metabolic flux or metabolite levels .
Transport and Distribution
This compound is transported and distributed within cells and tissues. This includes any transporters or binding proteins that it interacts with, as well as any effects on its localization or accumulation .
Subcellular Localization
This could include any targeting signals or post-translational modifications that direct it to specific compartments or organelles .
Preparation Methods
Synthetic Routes and Reaction Conditions
The synthesis of Acyclovir Acetate typically involves the reaction of guanine with 2-oxa-1,4-butanediol diacetate in the presence of a catalyst. One common method includes heating a mixture of guanine, hexamethyldisilazane, and ammonium sulfate to 115-118°C for 18 hours. This process forms a silylated intermediate, which is then reacted with 2-oxa-1,4-butanediol diacetate and trimethylsilyl triflate at 130°C for 4.5 hours. The final product is obtained by cooling the mixture and precipitating the compound .
Industrial Production Methods
Industrial production methods for this compound are similar to laboratory synthesis but are scaled up to accommodate larger quantities. The process involves careful control of reaction conditions to ensure high yield and purity. Chromatographic techniques are often employed to separate the desired product from isomeric impurities .
Chemical Reactions Analysis
Types of Reactions
Acyclovir Acetate undergoes various chemical reactions, including:
Substitution Reactions: The compound can participate in nucleophilic substitution reactions due to the presence of reactive functional groups.
Hydrolysis: The acetoxy group can be hydrolyzed to yield the corresponding hydroxyl derivative.
Oxidation and Reduction: While less common, the compound can undergo oxidation and reduction under specific conditions.
Common Reagents and Conditions
Nucleophilic Substitution: Common reagents include alkyl halides and bases.
Hydrolysis: Acidic or basic conditions can facilitate the hydrolysis of the acetoxy group.
Oxidation and Reduction: Specific oxidizing or reducing agents are used depending on the desired transformation.
Major Products Formed
Hydrolysis: Yields 9-(2-Hydroxyethoxymethyl)guanine.
Substitution: Depending on the nucleophile, various substituted derivatives can be formed.
Scientific Research Applications
Acyclovir Acetate has several applications in scientific research:
Chemistry: Used as an intermediate in the synthesis of antiviral nucleosides.
Biology: Studied for its potential antiviral activity against herpes viruses.
Medicine: Serves as a precursor in the production of antiviral drugs like acyclovir.
Industry: Employed in the large-scale synthesis of pharmaceutical compounds
Comparison with Similar Compounds
Similar Compounds
Acyclovir: A widely used antiviral drug with a similar structure.
Ganciclovir: Another antiviral agent with structural similarities.
Penciclovir: Shares a similar mechanism of action and is used to treat herpes infections.
Uniqueness
Acyclovir Acetate is unique due to its specific functional groups that allow for targeted chemical modifications. Its acetoxyethoxymethyl moiety provides distinct reactivity compared to other guanine derivatives, making it a valuable intermediate in pharmaceutical synthesis .
Properties
IUPAC Name |
2-[(2-amino-6-oxo-1H-purin-9-yl)methoxy]ethyl acetate | |
---|---|---|
Source | PubChem | |
URL | https://pubchem.ncbi.nlm.nih.gov | |
Description | Data deposited in or computed by PubChem | |
InChI |
InChI=1S/C10H13N5O4/c1-6(16)19-3-2-18-5-15-4-12-7-8(15)13-10(11)14-9(7)17/h4H,2-3,5H2,1H3,(H3,11,13,14,17) | |
Source | PubChem | |
URL | https://pubchem.ncbi.nlm.nih.gov | |
Description | Data deposited in or computed by PubChem | |
InChI Key |
DMHAXLGAKQREIL-UHFFFAOYSA-N | |
Source | PubChem | |
URL | https://pubchem.ncbi.nlm.nih.gov | |
Description | Data deposited in or computed by PubChem | |
Canonical SMILES |
CC(=O)OCCOCN1C=NC2=C1N=C(NC2=O)N | |
Source | PubChem | |
URL | https://pubchem.ncbi.nlm.nih.gov | |
Description | Data deposited in or computed by PubChem | |
Molecular Formula |
C10H13N5O4 | |
Source | PubChem | |
URL | https://pubchem.ncbi.nlm.nih.gov | |
Description | Data deposited in or computed by PubChem | |
DSSTOX Substance ID |
DTXSID50145470 | |
Record name | 9-(2-Acetoxyethoxymethyl)guanine | |
Source | EPA DSSTox | |
URL | https://comptox.epa.gov/dashboard/DTXSID50145470 | |
Description | DSSTox provides a high quality public chemistry resource for supporting improved predictive toxicology. | |
Molecular Weight |
267.24 g/mol | |
Source | PubChem | |
URL | https://pubchem.ncbi.nlm.nih.gov | |
Description | Data deposited in or computed by PubChem | |
CAS No. |
102728-64-3 | |
Record name | 9-(2-Acetoxyethoxymethyl)guanine | |
Source | ChemIDplus | |
URL | https://pubchem.ncbi.nlm.nih.gov/substance/?source=chemidplus&sourceid=0102728643 | |
Description | ChemIDplus is a free, web search system that provides access to the structure and nomenclature authority files used for the identification of chemical substances cited in National Library of Medicine (NLM) databases, including the TOXNET system. | |
Record name | 9-(2-Acetoxyethoxymethyl)guanine | |
Source | EPA DSSTox | |
URL | https://comptox.epa.gov/dashboard/DTXSID50145470 | |
Description | DSSTox provides a high quality public chemistry resource for supporting improved predictive toxicology. | |
Record name | 9-[[2-(Acetyloxy)ethoxy]methyl]-2-amino-1,9-dihydro-6H-purin-6-one | |
Source | European Chemicals Agency (ECHA) | |
URL | https://echa.europa.eu/information-on-chemicals | |
Description | The European Chemicals Agency (ECHA) is an agency of the European Union which is the driving force among regulatory authorities in implementing the EU's groundbreaking chemicals legislation for the benefit of human health and the environment as well as for innovation and competitiveness. | |
Explanation | Use of the information, documents and data from the ECHA website is subject to the terms and conditions of this Legal Notice, and subject to other binding limitations provided for under applicable law, the information, documents and data made available on the ECHA website may be reproduced, distributed and/or used, totally or in part, for non-commercial purposes provided that ECHA is acknowledged as the source: "Source: European Chemicals Agency, http://echa.europa.eu/". Such acknowledgement must be included in each copy of the material. ECHA permits and encourages organisations and individuals to create links to the ECHA website under the following cumulative conditions: Links can only be made to webpages that provide a link to the Legal Notice page. | |
Record name | 9-(2-ACETOXYETHOXYMETHYL)GUANINE | |
Source | FDA Global Substance Registration System (GSRS) | |
URL | https://gsrs.ncats.nih.gov/ginas/app/beta/substances/Y16CE349S3 | |
Description | The FDA Global Substance Registration System (GSRS) enables the efficient and accurate exchange of information on what substances are in regulated products. Instead of relying on names, which vary across regulatory domains, countries, and regions, the GSRS knowledge base makes it possible for substances to be defined by standardized, scientific descriptions. | |
Explanation | Unless otherwise noted, the contents of the FDA website (www.fda.gov), both text and graphics, are not copyrighted. They are in the public domain and may be republished, reprinted and otherwise used freely by anyone without the need to obtain permission from FDA. Credit to the U.S. Food and Drug Administration as the source is appreciated but not required. | |
Retrosynthesis Analysis
AI-Powered Synthesis Planning: Our tool employs the Template_relevance Pistachio, Template_relevance Bkms_metabolic, Template_relevance Pistachio_ringbreaker, Template_relevance Reaxys, Template_relevance Reaxys_biocatalysis model, leveraging a vast database of chemical reactions to predict feasible synthetic routes.
One-Step Synthesis Focus: Specifically designed for one-step synthesis, it provides concise and direct routes for your target compounds, streamlining the synthesis process.
Accurate Predictions: Utilizing the extensive PISTACHIO, BKMS_METABOLIC, PISTACHIO_RINGBREAKER, REAXYS, REAXYS_BIOCATALYSIS database, our tool offers high-accuracy predictions, reflecting the latest in chemical research and data.
Strategy Settings
Precursor scoring | Relevance Heuristic |
---|---|
Min. plausibility | 0.01 |
Model | Template_relevance |
Template Set | Pistachio/Bkms_metabolic/Pistachio_ringbreaker/Reaxys/Reaxys_biocatalysis |
Top-N result to add to graph | 6 |
Feasible Synthetic Routes
Q1: What is the significance of the platinum complexes formed with 9-(2-Acetoxyethoxymethyl)guanine and how was their structure confirmed?
A1: The research highlights the synthesis and characterization of platinum complexes with 9-(2-Acetoxyethoxymethyl)guanine (monoacetylacyclovir) and related guanine derivatives []. These complexes are of interest due to the potential antitumor activity of platinum-based compounds and the biological relevance of modified guanine nucleosides. The crystal structure of the complex trans-[9-(2-acetoxyethoxymethyl)guanine-κN7]dichloro(η-ethylene)platinum(II) was determined using X-ray crystallography, revealing square planar coordination around the platinum atom involving the ethylene ligand, two chloride ions, and the N7 nitrogen of the guanine base []. This structural information provides valuable insights into the mode of binding and potential interactions of these complexes with biological targets.
Q2: Can you describe a synthetic route for producing 9-(2-Acetoxyethoxymethyl)guanine?
A2: One of the research articles outlines a method to synthesize a related compound, 2-[[2-(acetylamino)-6-oxo-1,6-dihydro-7H-purin-7-yl]methoxyl]ethyl acetate, which can be hydrolyzed to obtain 2-amino-7-[(2-hydroxyehoxy)methyl]-1,7-dihydro-6H-purin-6-one (also known as acyclovir) []. While the synthesis of 9-(2-Acetoxyethoxymethyl)guanine itself is not directly described, it's plausible that a similar condensation reaction using appropriately protected starting materials could be employed. Further investigation and optimization of reaction conditions might be needed to achieve the desired product.
Disclaimer and Information on In-Vitro Research Products
Please be aware that all articles and product information presented on BenchChem are intended solely for informational purposes. The products available for purchase on BenchChem are specifically designed for in-vitro studies, which are conducted outside of living organisms. In-vitro studies, derived from the Latin term "in glass," involve experiments performed in controlled laboratory settings using cells or tissues. It is important to note that these products are not categorized as medicines or drugs, and they have not received approval from the FDA for the prevention, treatment, or cure of any medical condition, ailment, or disease. We must emphasize that any form of bodily introduction of these products into humans or animals is strictly prohibited by law. It is essential to adhere to these guidelines to ensure compliance with legal and ethical standards in research and experimentation.