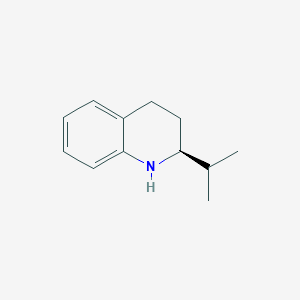
(S)-2-isopropyl-1,2,3,4-tetrahydroquinoline
- Click on QUICK INQUIRY to receive a quote from our team of experts.
- With the quality product at a COMPETITIVE price, you can focus more on your research.
Overview
Description
(S)-2-Isopropyl-1,2,3,4-tetrahydroquinoline is a chiral organic compound belonging to the tetrahydroquinoline family This compound is characterized by its unique structure, which includes an isopropyl group attached to the second carbon of the tetrahydroquinoline ring The (S)-enantiomer indicates that the compound has a specific three-dimensional arrangement, making it optically active
Preparation Methods
Synthetic Routes and Reaction Conditions: The synthesis of (S)-2-isopropyl-1,2,3,4-tetrahydroquinoline typically involves the catalytic hydrogenation of quinoline derivatives. One common method includes the use of chiral catalysts to ensure the production of the (S)-enantiomer. The reaction conditions often involve hydrogen gas at elevated pressures and temperatures, along with a suitable solvent such as ethanol or methanol.
Industrial Production Methods: In an industrial setting, the production of this compound may involve continuous flow reactors to optimize yield and efficiency. The use of chiral catalysts in large-scale hydrogenation processes ensures the selective production of the desired enantiomer. Additionally, purification techniques such as crystallization and chromatography are employed to achieve high enantiomeric purity.
Chemical Reactions Analysis
Types of Reactions: (S)-2-Isopropyl-1,2,3,4-tetrahydroquinoline undergoes various chemical reactions, including:
Oxidation: This compound can be oxidized to form quinoline derivatives using oxidizing agents such as potassium permanganate or chromium trioxide.
Reduction: Reduction reactions can further hydrogenate the compound to produce fully saturated derivatives.
Substitution: Nucleophilic substitution reactions can occur at the nitrogen atom or the aromatic ring, leading to the formation of various substituted derivatives.
Common Reagents and Conditions:
Oxidation: Potassium permanganate in acidic or neutral conditions.
Reduction: Hydrogen gas with palladium on carbon as a catalyst.
Substitution: Alkyl halides or acyl chlorides in the presence of a base such as sodium hydride.
Major Products:
Oxidation: Quinoline derivatives.
Reduction: Fully saturated tetrahydroquinoline derivatives.
Substitution: N-alkyl or N-acyl tetrahydroquinoline derivatives.
Scientific Research Applications
(S)-2-Isopropyl-1,2,3,4-tetrahydroquinoline has a wide range of applications in scientific research:
Chemistry: It serves as a building block for the synthesis of more complex molecules and as a chiral ligand in asymmetric synthesis.
Biology: The compound is studied for its potential biological activities, including antimicrobial and anticancer properties.
Medicine: It is explored as a potential pharmaceutical intermediate for the development of drugs targeting neurological disorders and cardiovascular diseases.
Industry: The compound is used in the production of agrochemicals and as an additive in materials science for the development of advanced polymers and coatings.
Mechanism of Action
The mechanism of action of (S)-2-isopropyl-1,2,3,4-tetrahydroquinoline varies depending on its application. In biological systems, it may interact with specific molecular targets such as enzymes or receptors, modulating their activity. For example, it may inhibit certain enzymes involved in disease pathways, leading to therapeutic effects. The compound’s chiral nature allows it to fit into specific binding sites, enhancing its selectivity and potency.
Comparison with Similar Compounds
2-Isopropyl-1,2,3,4-tetrahydroquinoline: The non-chiral version of the compound.
2-Methyl-1,2,3,4-tetrahydroquinoline: A similar compound with a methyl group instead of an isopropyl group.
2-Ethyl-1,2,3,4-tetrahydroquinoline: A compound with an ethyl group at the same position.
Uniqueness: (S)-2-Isopropyl-1,2,3,4-tetrahydroquinoline is unique due to its chiral nature, which imparts specific optical activity and potential for enantioselective interactions. This makes it particularly valuable in asymmetric synthesis and pharmaceutical applications where enantiomeric purity is crucial.
Biological Activity
(S)-2-Isopropyl-1,2,3,4-tetrahydroquinoline is a nitrogen-containing heterocyclic compound that has garnered attention for its diverse biological activities. This compound features a saturated quinoline structure that contributes to its pharmacological properties. Research indicates that it exhibits neuroprotective effects, antimicrobial activity, and potential applications in treating various diseases.
Chemical Structure and Properties
This compound has the following molecular formula: C12H15N. The presence of the isopropyl group enhances its lipophilicity, which may influence its biological interactions and therapeutic efficacy. The compound's structural characteristics allow for various chemical modifications to enhance its biological activity.
Neuroprotective Effects
Research suggests that this compound may protect neuronal cells from damage associated with neurodegenerative diseases. It has been shown to inhibit oxidative stress and reduce apoptosis in neuronal cell lines, indicating its potential as a neuroprotective agent .
Antimicrobial Activity
This compound also exhibits antimicrobial properties against various bacterial strains. Studies have demonstrated its effectiveness against Gram-positive and Gram-negative bacteria, making it a candidate for further investigation in the development of new antimicrobial agents .
Antiviral Properties
There is emerging evidence that quinoline derivatives, including this compound, possess antiviral activities. They have been tested against several viral strains with promising results. The mechanism of action may involve interference with viral replication processes .
The biological activities of this compound can be attributed to its interaction with various biological targets. Interaction studies indicate that it binds to specific receptors or enzymes involved in disease pathways. For instance:
- Neuroprotection : It may modulate signaling pathways associated with neuroprotection.
- Antimicrobial Action : Its binding affinity to bacterial enzymes could inhibit cell wall synthesis or disrupt metabolic pathways.
- Antiviral Mechanism : Potential inhibition of viral polymerases or proteases has been suggested as a mechanism for its antiviral effects .
Synthesis Methods
Several synthetic routes have been developed for this compound:
- Skraup Reaction : A classical method involving the condensation of aniline derivatives with carbonyl compounds.
- Combes Reaction : Another synthetic approach that utilizes specific reagents to form the tetrahydroquinoline structure efficiently.
These methods highlight the versatility in synthesizing this compound and its derivatives .
Comparative Analysis
To better understand the uniqueness of this compound compared to similar compounds, the following table summarizes some related compounds and their notable properties:
Compound Name | Structure Type | Notable Properties |
---|---|---|
1,2,3,4-Tetrahydroquinoline | Tetrahydroquinoline | Basic scaffold; exhibits neuroprotective activity |
1-Methyl-1,2,3,4-tetrahydroquinoline | Methylated derivative | Enhanced lipophilicity; potential CNS activity |
1-Benzyl-1,2,3,4-tetrahydroquinoline | Benzyl derivative | Exhibits enhanced antimicrobial properties compared to basic THQ |
The unique presence of the isopropyl group in this compound contributes to its distinct chemical behavior and biological activity compared to these similar compounds.
Case Studies
In recent studies focused on neurodegenerative diseases such as Alzheimer's and Parkinson's disease:
- Case Study 1 : In vitro studies demonstrated that this compound reduced neuronal cell death induced by amyloid-beta peptides.
- Case Study 2 : Animal models treated with this compound showed improved cognitive function and reduced neuroinflammation markers compared to control groups.
These findings underscore the potential therapeutic applications of this compound in neuroprotection and possibly in other areas such as infectious disease management .
Properties
Molecular Formula |
C12H17N |
---|---|
Molecular Weight |
175.27 g/mol |
IUPAC Name |
(2S)-2-propan-2-yl-1,2,3,4-tetrahydroquinoline |
InChI |
InChI=1S/C12H17N/c1-9(2)11-8-7-10-5-3-4-6-12(10)13-11/h3-6,9,11,13H,7-8H2,1-2H3/t11-/m0/s1 |
InChI Key |
QTMOXLMMSRXXGQ-NSHDSACASA-N |
Isomeric SMILES |
CC(C)[C@@H]1CCC2=CC=CC=C2N1 |
Canonical SMILES |
CC(C)C1CCC2=CC=CC=C2N1 |
Origin of Product |
United States |
Disclaimer and Information on In-Vitro Research Products
Please be aware that all articles and product information presented on BenchChem are intended solely for informational purposes. The products available for purchase on BenchChem are specifically designed for in-vitro studies, which are conducted outside of living organisms. In-vitro studies, derived from the Latin term "in glass," involve experiments performed in controlled laboratory settings using cells or tissues. It is important to note that these products are not categorized as medicines or drugs, and they have not received approval from the FDA for the prevention, treatment, or cure of any medical condition, ailment, or disease. We must emphasize that any form of bodily introduction of these products into humans or animals is strictly prohibited by law. It is essential to adhere to these guidelines to ensure compliance with legal and ethical standards in research and experimentation.