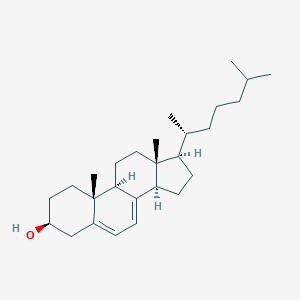
7-Dehydrocholesterol
Overview
Description
Synthesis Analysis
7-DHC is synthesized from lathosterol by the enzyme lathosterol oxidase (lathosterol 5-desaturase) . This is the next-to-last step of cholesterol biosynthesis . Defective synthesis results in the human inherited disorder lathosterolosis resembling Smith–Lemli–Opitz syndrome .Molecular Structure Analysis
The molecular formula of 7-DHC is C27H44O . Its IUPAC name is (3S,9S,10R,13R,14R,17R)-10,13-dimethyl-17-[(2R)-6-methylheptan-2-yl]-2,3,4,9,11,12,14,15,16,17-decahydro-1H-cyclopenta[a]phenanthren-3-ol .Chemical Reactions Analysis
7-DHC is known to react with 4-phenyl-1,2,4-triazoline-3,5-dione (PTAD) in a Diels-Alder cycloaddition reaction . This reaction is used for the detection of 7-DHC in various biological tissues and fluids .Physical And Chemical Properties Analysis
7-DHC has a molecular weight of 384.6 g/mol . It is a 3beta-sterol, a cholestanoid, a Delta(5),Delta(7)-sterol, and a 3beta-hydroxy-Delta(5)-steroid .Scientific Research Applications
Antioxidant Properties and Ferroptosis Prevention
7-Dehydrocholesterol (7-DHC) has been identified as a lipid-soluble antioxidant that protects cells from ferroptosis . This cell death pathway is triggered by iron-catalyzed phospholipid peroxidation and plays a significant role in various degenerative diseases. The unique structure of 7-DHC, with its conjugated double bonds, allows it to reduce lipid peroxyl radicals, including those present in cell membranes, which are essential to drive ferroptosis .
Cancer Treatment
The accumulation of 7-DHC in cells, due to the inhibition of the enzyme 7-dehydrocholesterol reductase (DHCR7), renders cells resistant to ferroptosis . This has implications for cancer treatment, as certain cancer cells that are resistant to conventional therapies are notably vulnerable to ferroptosis. Targeting ferroptosis, either by stimulating or inhibiting it, emerges as a promising approach for treating cancers that evade standard treatments .
Vitamin D3 Synthesis
7-DHC is a precursor to vitamin D3, which can be converted to the active form after UV irradiation . Vitamin D3 is crucial for preventing and treating bone diseases like rickets, improving immunity, and reducing cardiovascular disease risk. Enhancing the supply of 7-DHC is vital for the production of steroids, including vitamin D3 .
Treatment of Liver and Kidney Diseases
7-DHC is also a precursor for 25-hydroxyvitamin D3 production, the active form of vitamin D3 widely used to treat patients with severe liver and kidney disease . Improving the production of 7-DHC could lead to better treatment options for these conditions.
Industrial Production and Steroid Development
Reengineering the biosynthesis of 7-DHC in organisms like Saccharomyces cerevisiae has shown significant promise for industrial production . This advancement is of great significance for the development of medically important steroids and could lead to more cost-effective production methods.
Microbial Production of Medicinally Important Agents
The microbial production of 7-DHC has been explored as a means to create medicinally important agents. By modifying the biosynthesis pathway in microorganisms, researchers have been able to increase the yield of 7-DHC, which is crucial for the production of various steroidal drugs .
Biomedical Applications
The protective function of 7-DHC against cell death opens up new prospects for improving the treatment of diseases associated with ferroptosis . This includes the potential development of new inhibitors that target cholesterol biosynthesis, which are already established in medical practice .
Feedstuff and Nutraceutical Uses
Due to its role as a precursor to vitamin D3, 7-DHC is also widely used in feedstuffs to improve the health and productivity of livestock. Additionally, it has applications in nutraceuticals, providing health benefits beyond basic nutrition .
Mechanism of Action
Target of Action
7-Dehydrocholesterol (7-DHC) is a sterol produced through the cholesterol biosynthetic pathway . It primarily targets cells to protect them from a cell death pathway known as ferroptosis . Ferroptosis is characterized by iron-catalyzed accumulation of phospholipid peroxides and plays a critical role in various degenerative diseases that cause organ damage .
Mode of Action
7-DHC interacts with its targets by functioning as a lipid-soluble antioxidant . It shields (phospho)lipids from autoxidation and subsequent fragmentation . This unique structure, combined with the physical presence of 7-DHC in membranes, allows 7-DHC to reduce lipid peroxyl radicals, including phospholipid peroxyl radicals present in membranes, which are essential to drive ferroptosis .
Biochemical Pathways
7-DHC is synthesized from lathosterol by the enzyme lathosterol oxidase (lathosterol 5-desaturase), which is the next-to-last step of cholesterol biosynthesis . Inhibiting 7-dehydrocholesterol reductase (DHCR7), the last enzyme involved in cholesterol biosynthesis, leads to the accumulation of 7-DHC . Both research teams identified DHCR7, which catalyzes the conversion of 7-DHC to cholesterol, as a pivotal regulator for ferroptosis .
Pharmacokinetics
It’s known that 7-dhc has a high lipophilicity, with a predicted log p value of 902 . This suggests that it may have good membrane permeability and could potentially influence its absorption, distribution, metabolism, and excretion (ADME) properties.
Result of Action
The accumulation of 7-DHC protects cells from ferroptosis . By inhibiting the production of 7-DHC, ferroptosis can be induced, and tumor growth can be suppressed . On the other hand, increasing the level of 7-DHC by inhibiting DHCR7 can promote cancer metastasis and slow the progression of renal ischemia-reperfusion injury .
Action Environment
The action of 7-DHC can be influenced by environmental factors such as ultraviolet (UV) light. Upon exposure to UV-B rays in sunlight, 7-DHC is converted into vitamin D3 via previtamin D3 as an intermediate isomer . This suggests that the action, efficacy, and stability of 7-DHC can be influenced by environmental factors such as exposure to sunlight.
Safety and Hazards
Future Directions
Future research should explore the effects of 7-DHC accumulation on tumor development and the potential impacts of drugs that can inhibit the DHCR7 enzyme, which can lead to elevated plasma levels of 7-DHC . Pharmacological manipulation of 7-DHC levels shows promise as a therapeutic strategy for cancer and ischemia-reperfusion injury .
properties
IUPAC Name |
(3S,9S,10R,13R,14R,17R)-10,13-dimethyl-17-[(2R)-6-methylheptan-2-yl]-2,3,4,9,11,12,14,15,16,17-decahydro-1H-cyclopenta[a]phenanthren-3-ol | |
---|---|---|
Source | PubChem | |
URL | https://pubchem.ncbi.nlm.nih.gov | |
Description | Data deposited in or computed by PubChem | |
InChI |
InChI=1S/C27H44O/c1-18(2)7-6-8-19(3)23-11-12-24-22-10-9-20-17-21(28)13-15-26(20,4)25(22)14-16-27(23,24)5/h9-10,18-19,21,23-25,28H,6-8,11-17H2,1-5H3/t19-,21+,23-,24+,25+,26+,27-/m1/s1 | |
Source | PubChem | |
URL | https://pubchem.ncbi.nlm.nih.gov | |
Description | Data deposited in or computed by PubChem | |
InChI Key |
UCTLRSWJYQTBFZ-DDPQNLDTSA-N | |
Source | PubChem | |
URL | https://pubchem.ncbi.nlm.nih.gov | |
Description | Data deposited in or computed by PubChem | |
Canonical SMILES |
CC(C)CCCC(C)C1CCC2C1(CCC3C2=CC=C4C3(CCC(C4)O)C)C | |
Source | PubChem | |
URL | https://pubchem.ncbi.nlm.nih.gov | |
Description | Data deposited in or computed by PubChem | |
Isomeric SMILES |
C[C@H](CCCC(C)C)[C@H]1CC[C@@H]2[C@@]1(CC[C@H]3C2=CC=C4[C@@]3(CC[C@@H](C4)O)C)C | |
Source | PubChem | |
URL | https://pubchem.ncbi.nlm.nih.gov | |
Description | Data deposited in or computed by PubChem | |
Molecular Formula |
C27H44O | |
Source | PubChem | |
URL | https://pubchem.ncbi.nlm.nih.gov | |
Description | Data deposited in or computed by PubChem | |
DSSTOX Substance ID |
DTXSID20861933 | |
Record name | 7,8-Didehydrocholesterol | |
Source | EPA DSSTox | |
URL | https://comptox.epa.gov/dashboard/DTXSID20861933 | |
Description | DSSTox provides a high quality public chemistry resource for supporting improved predictive toxicology. | |
Molecular Weight |
384.6 g/mol | |
Source | PubChem | |
URL | https://pubchem.ncbi.nlm.nih.gov | |
Description | Data deposited in or computed by PubChem | |
Physical Description |
Solid | |
Record name | 7-Dehydrocholesterol | |
Source | Human Metabolome Database (HMDB) | |
URL | http://www.hmdb.ca/metabolites/HMDB0000032 | |
Description | The Human Metabolome Database (HMDB) is a freely available electronic database containing detailed information about small molecule metabolites found in the human body. | |
Explanation | HMDB is offered to the public as a freely available resource. Use and re-distribution of the data, in whole or in part, for commercial purposes requires explicit permission of the authors and explicit acknowledgment of the source material (HMDB) and the original publication (see the HMDB citing page). We ask that users who download significant portions of the database cite the HMDB paper in any resulting publications. | |
Solubility |
1 mg/mL | |
Record name | 7-Dehydrocholesterol | |
Source | Human Metabolome Database (HMDB) | |
URL | http://www.hmdb.ca/metabolites/HMDB0000032 | |
Description | The Human Metabolome Database (HMDB) is a freely available electronic database containing detailed information about small molecule metabolites found in the human body. | |
Explanation | HMDB is offered to the public as a freely available resource. Use and re-distribution of the data, in whole or in part, for commercial purposes requires explicit permission of the authors and explicit acknowledgment of the source material (HMDB) and the original publication (see the HMDB citing page). We ask that users who download significant portions of the database cite the HMDB paper in any resulting publications. | |
Product Name |
7-Dehydrocholesterol | |
CAS RN |
434-16-2 | |
Record name | 7-Dehydrocholesterol | |
Source | CAS Common Chemistry | |
URL | https://commonchemistry.cas.org/detail?cas_rn=434-16-2 | |
Description | CAS Common Chemistry is an open community resource for accessing chemical information. Nearly 500,000 chemical substances from CAS REGISTRY cover areas of community interest, including common and frequently regulated chemicals, and those relevant to high school and undergraduate chemistry classes. This chemical information, curated by our expert scientists, is provided in alignment with our mission as a division of the American Chemical Society. | |
Explanation | The data from CAS Common Chemistry is provided under a CC-BY-NC 4.0 license, unless otherwise stated. | |
Record name | 7-Dehydrocholesterol | |
Source | ChemIDplus | |
URL | https://pubchem.ncbi.nlm.nih.gov/substance/?source=chemidplus&sourceid=0000434162 | |
Description | ChemIDplus is a free, web search system that provides access to the structure and nomenclature authority files used for the identification of chemical substances cited in National Library of Medicine (NLM) databases, including the TOXNET system. | |
Record name | Cholesta-5,7-dien-3-ol, (3.beta.)- | |
Source | EPA Chemicals under the TSCA | |
URL | https://www.epa.gov/chemicals-under-tsca | |
Description | EPA Chemicals under the Toxic Substances Control Act (TSCA) collection contains information on chemicals and their regulations under TSCA, including non-confidential content from the TSCA Chemical Substance Inventory and Chemical Data Reporting. | |
Record name | 7,8-Didehydrocholesterol | |
Source | EPA DSSTox | |
URL | https://comptox.epa.gov/dashboard/DTXSID20861933 | |
Description | DSSTox provides a high quality public chemistry resource for supporting improved predictive toxicology. | |
Record name | 7,8-didehydrocholesterol | |
Source | European Chemicals Agency (ECHA) | |
URL | https://echa.europa.eu/substance-information/-/substanceinfo/100.006.456 | |
Description | The European Chemicals Agency (ECHA) is an agency of the European Union which is the driving force among regulatory authorities in implementing the EU's groundbreaking chemicals legislation for the benefit of human health and the environment as well as for innovation and competitiveness. | |
Explanation | Use of the information, documents and data from the ECHA website is subject to the terms and conditions of this Legal Notice, and subject to other binding limitations provided for under applicable law, the information, documents and data made available on the ECHA website may be reproduced, distributed and/or used, totally or in part, for non-commercial purposes provided that ECHA is acknowledged as the source: "Source: European Chemicals Agency, http://echa.europa.eu/". Such acknowledgement must be included in each copy of the material. ECHA permits and encourages organisations and individuals to create links to the ECHA website under the following cumulative conditions: Links can only be made to webpages that provide a link to the Legal Notice page. | |
Record name | 7-DEHYDROCHOLESTEROL | |
Source | FDA Global Substance Registration System (GSRS) | |
URL | https://gsrs.ncats.nih.gov/ginas/app/beta/substances/BK1IU07GKF | |
Description | The FDA Global Substance Registration System (GSRS) enables the efficient and accurate exchange of information on what substances are in regulated products. Instead of relying on names, which vary across regulatory domains, countries, and regions, the GSRS knowledge base makes it possible for substances to be defined by standardized, scientific descriptions. | |
Explanation | Unless otherwise noted, the contents of the FDA website (www.fda.gov), both text and graphics, are not copyrighted. They are in the public domain and may be republished, reprinted and otherwise used freely by anyone without the need to obtain permission from FDA. Credit to the U.S. Food and Drug Administration as the source is appreciated but not required. | |
Record name | 7-Dehydrocholesterol | |
Source | Human Metabolome Database (HMDB) | |
URL | http://www.hmdb.ca/metabolites/HMDB0000032 | |
Description | The Human Metabolome Database (HMDB) is a freely available electronic database containing detailed information about small molecule metabolites found in the human body. | |
Explanation | HMDB is offered to the public as a freely available resource. Use and re-distribution of the data, in whole or in part, for commercial purposes requires explicit permission of the authors and explicit acknowledgment of the source material (HMDB) and the original publication (see the HMDB citing page). We ask that users who download significant portions of the database cite the HMDB paper in any resulting publications. | |
Melting Point |
150.5 °C | |
Record name | 7-Dehydrocholesterol | |
Source | Human Metabolome Database (HMDB) | |
URL | http://www.hmdb.ca/metabolites/HMDB0000032 | |
Description | The Human Metabolome Database (HMDB) is a freely available electronic database containing detailed information about small molecule metabolites found in the human body. | |
Explanation | HMDB is offered to the public as a freely available resource. Use and re-distribution of the data, in whole or in part, for commercial purposes requires explicit permission of the authors and explicit acknowledgment of the source material (HMDB) and the original publication (see the HMDB citing page). We ask that users who download significant portions of the database cite the HMDB paper in any resulting publications. | |
Retrosynthesis Analysis
AI-Powered Synthesis Planning: Our tool employs the Template_relevance Pistachio, Template_relevance Bkms_metabolic, Template_relevance Pistachio_ringbreaker, Template_relevance Reaxys, Template_relevance Reaxys_biocatalysis model, leveraging a vast database of chemical reactions to predict feasible synthetic routes.
One-Step Synthesis Focus: Specifically designed for one-step synthesis, it provides concise and direct routes for your target compounds, streamlining the synthesis process.
Accurate Predictions: Utilizing the extensive PISTACHIO, BKMS_METABOLIC, PISTACHIO_RINGBREAKER, REAXYS, REAXYS_BIOCATALYSIS database, our tool offers high-accuracy predictions, reflecting the latest in chemical research and data.
Strategy Settings
Precursor scoring | Relevance Heuristic |
---|---|
Min. plausibility | 0.01 |
Model | Template_relevance |
Template Set | Pistachio/Bkms_metabolic/Pistachio_ringbreaker/Reaxys/Reaxys_biocatalysis |
Top-N result to add to graph | 6 |
Feasible Synthetic Routes
Q & A
Q1: What is 7-dehydrocholesterol and what is its role in cholesterol biosynthesis?
A: 7-Dehydrocholesterol is a sterol and the immediate precursor to cholesterol in the Kandutsch-Russell pathway of cholesterol biosynthesis []. The enzyme 7-dehydrocholesterol reductase (DHCR7) catalyzes the final step, reducing 7-dehydrocholesterol to cholesterol [, , , , ].
Q2: What is Smith-Lemli-Opitz syndrome (SLOS) and how is it related to 7-dehydrocholesterol?
A: Smith-Lemli-Opitz syndrome is an autosomal recessive disorder characterized by multiple congenital anomalies, mental retardation, and growth defects [, , ]. It arises from mutations in the DHCR7 gene, leading to a deficiency of cholesterol and an accumulation of 7-dehydrocholesterol in tissues and fluids [, , , , , , , , , ].
Q3: How is SLOS diagnosed and what are the typical biochemical findings?
A: SLOS can be diagnosed by detecting markedly increased concentrations of 7-dehydrocholesterol in plasma, typically using gas chromatography-mass spectrometry [, , , , ]. Alternatively, a deficiency in the conversion of 7-dehydrocholesterol to cholesterol can be demonstrated in cultured fibroblasts from patients [, ]. Prenatal diagnosis is also possible by quantifying sterol levels in amniotic fluid [].
Q4: What is the significance of the different biochemical variants observed in SLOS patients?
A: Studies have shown that the severity of SLOS can vary, with some patients exhibiting more severe clinical symptoms than others []. These clinical differences are often reflected in the degree to which 7-dehydrocholesterol accumulates and cholesterol synthesis is impaired []. These biochemical variations likely result from different mutations in the DHCR7 gene, leading to varying degrees of enzyme deficiency [].
Q5: Does 7-dehydrocholesterol itself contribute to the pathogenesis of SLOS, or is it solely the cholesterol deficiency?
A: While cholesterol deficiency undoubtedly plays a major role in SLOS pathology, evidence suggests that accumulated 7-dehydrocholesterol might also exert toxic effects [, , , ]. For instance, 7-dehydrocholesterol is more susceptible to oxidation than cholesterol, and its oxidized derivatives have been shown to impair embryonic growth in animal models [, , ].
Q6: What is the connection between 7-dehydrocholesterol and vitamin D synthesis?
A: 7-Dehydrocholesterol serves as the precursor for vitamin D3 synthesis in the skin [, , , , , , , ]. Upon exposure to UVB radiation, 7-dehydrocholesterol undergoes photolysis to form previtamin D3, which subsequently isomerizes to vitamin D3 [, , , , , , , ].
Q7: Can altering 7-dehydrocholesterol levels impact vitamin D production?
A: Yes, factors influencing 7-dehydrocholesterol availability can affect vitamin D synthesis. For example, long-term exposure to ultraviolet radiation can increase 7-dehydrocholesterol levels in the skin, potentially enhancing vitamin D3 production [, ]. Conversely, inhibiting DHCR7 activity can also increase 7-dehydrocholesterol levels, leading to elevated vitamin D levels, as observed in some studies [, ].
Q8: How do seasonal changes and latitude affect vitamin D synthesis from 7-dehydrocholesterol?
A: The amount of UVB radiation reaching the Earth's surface varies significantly with season and latitude. During winter months, particularly at higher latitudes, insufficient UVB radiation reaches the skin to effectively convert 7-dehydrocholesterol to vitamin D3 []. This highlights the importance of dietary vitamin D intake during these periods.
Q9: What are the potential therapeutic applications of modulating 7-dehydrocholesterol metabolism?
A: Manipulating 7-dehydrocholesterol metabolism holds promise for various therapeutic applications. For instance, inhibiting DHCR7 could be a potential strategy for treating hyperlipidemia, as it can lead to decreased cholesterol levels [, , ]. Additionally, increasing 7-dehydrocholesterol availability could enhance vitamin D3 production, potentially benefiting individuals with vitamin D deficiency or related disorders [, , ].
Disclaimer and Information on In-Vitro Research Products
Please be aware that all articles and product information presented on BenchChem are intended solely for informational purposes. The products available for purchase on BenchChem are specifically designed for in-vitro studies, which are conducted outside of living organisms. In-vitro studies, derived from the Latin term "in glass," involve experiments performed in controlled laboratory settings using cells or tissues. It is important to note that these products are not categorized as medicines or drugs, and they have not received approval from the FDA for the prevention, treatment, or cure of any medical condition, ailment, or disease. We must emphasize that any form of bodily introduction of these products into humans or animals is strictly prohibited by law. It is essential to adhere to these guidelines to ensure compliance with legal and ethical standards in research and experimentation.