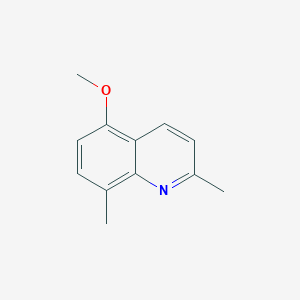
5-Methoxy-2,8-dimethylquinoline
- Click on QUICK INQUIRY to receive a quote from our team of experts.
- With the quality product at a COMPETITIVE price, you can focus more on your research.
Overview
Description
5-Methoxy-2,8-dimethylquinoline is a derivative of quinoline, a heterocyclic aromatic organic compound Quinoline and its derivatives are known for their diverse biological activities and are used in various fields such as medicinal chemistry, organic synthesis, and materials science
Preparation Methods
Synthetic Routes and Reaction Conditions
5-Methoxy-2,8-dimethylquinoline can be synthesized through several methods. One common approach involves the Skraup synthesis, which is a classical method for producing quinoline derivatives. This method typically involves the reaction of aniline derivatives with glycerol and sulfuric acid in the presence of an oxidizing agent such as nitrobenzene .
Another method involves the Friedländer synthesis, which involves the condensation of 2-aminobenzophenone with an aldehyde or ketone under acidic or basic conditions . Additionally, microwave-assisted synthesis has been employed to produce quinoline derivatives efficiently and with high yields .
Industrial Production Methods
Industrial production of this compound may involve large-scale application of the aforementioned synthetic routes. The use of continuous flow reactors and optimization of reaction conditions can enhance the efficiency and yield of the production process. Green chemistry approaches, such as solvent-free reactions and the use of recyclable catalysts, are also being explored to make the production process more sustainable .
Chemical Reactions Analysis
Types of Reactions
5-Methoxy-2,8-dimethylquinoline undergoes various chemical reactions, including:
Oxidation: The compound can be oxidized to form quinoline N-oxide derivatives.
Reduction: Reduction reactions can convert the quinoline ring to tetrahydroquinoline derivatives.
Substitution: Electrophilic and nucleophilic substitution reactions can introduce different functional groups into the quinoline ring.
Common Reagents and Conditions
Common reagents used in these reactions include oxidizing agents like hydrogen peroxide for oxidation, reducing agents like sodium borohydride for reduction, and halogenating agents for substitution reactions. Reaction conditions such as temperature, solvent, and catalyst choice play a crucial role in determining the reaction outcome .
Major Products Formed
The major products formed from these reactions include quinoline N-oxides, tetrahydroquinoline derivatives, and various substituted quinolines. These products have significant applications in medicinal chemistry and materials science .
Scientific Research Applications
5-Methoxy-2,8-dimethylquinoline has several scientific research applications:
Medicinal Chemistry: It is used as a scaffold for designing drugs with anticancer, antimicrobial, and anti-inflammatory activities.
Organic Synthesis: The compound serves as an intermediate in the synthesis of more complex molecules and natural products.
Materials Science: Quinoline derivatives are used in the development of organic light-emitting diodes (OLEDs) and other electronic materials.
Mechanism of Action
The mechanism of action of 5-Methoxy-2,8-dimethylquinoline involves its interaction with various molecular targets. For instance, quinoline derivatives have been shown to inhibit enzymes such as topoisomerases and kinases, which are involved in DNA replication and cell signaling pathways . The presence of the methoxy group may enhance the compound’s ability to interact with these targets, leading to its biological effects.
Comparison with Similar Compounds
Similar Compounds
Similar compounds to 5-Methoxy-2,8-dimethylquinoline include:
Quinoline: The parent compound with a simpler structure.
2-Methylquinoline: A derivative with a single methyl group.
5-Methoxyquinoline: A derivative with a methoxy group at the 5-position.
Uniqueness
This compound is unique due to the presence of both methoxy and methyl groups, which can enhance its chemical reactivity and biological activity. This combination of functional groups may provide a synergistic effect, making it a valuable compound for various applications .
Properties
Molecular Formula |
C12H13NO |
---|---|
Molecular Weight |
187.24 g/mol |
IUPAC Name |
5-methoxy-2,8-dimethylquinoline |
InChI |
InChI=1S/C12H13NO/c1-8-4-7-11(14-3)10-6-5-9(2)13-12(8)10/h4-7H,1-3H3 |
InChI Key |
HSTDUUIQCVOQEB-UHFFFAOYSA-N |
Canonical SMILES |
CC1=C2C(=C(C=C1)OC)C=CC(=N2)C |
Origin of Product |
United States |
Disclaimer and Information on In-Vitro Research Products
Please be aware that all articles and product information presented on BenchChem are intended solely for informational purposes. The products available for purchase on BenchChem are specifically designed for in-vitro studies, which are conducted outside of living organisms. In-vitro studies, derived from the Latin term "in glass," involve experiments performed in controlled laboratory settings using cells or tissues. It is important to note that these products are not categorized as medicines or drugs, and they have not received approval from the FDA for the prevention, treatment, or cure of any medical condition, ailment, or disease. We must emphasize that any form of bodily introduction of these products into humans or animals is strictly prohibited by law. It is essential to adhere to these guidelines to ensure compliance with legal and ethical standards in research and experimentation.