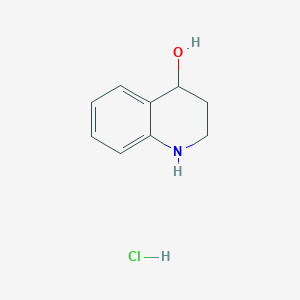
1,2,3,4-Tetrahydroquinolin-4-ol hydrochloride
- Click on QUICK INQUIRY to receive a quote from our team of experts.
- With the quality product at a COMPETITIVE price, you can focus more on your research.
Overview
Description
1,2,3,4-Tetrahydroquinolin-4-ol hydrochloride is a chemical compound belonging to the class of tetrahydroquinolines. These compounds are known for their diverse biological activities and are widely studied in medicinal chemistry. The compound has a molecular formula of C9H11NO·HCl and is often used in various chemical and pharmaceutical research applications .
Preparation Methods
Synthetic Routes and Reaction Conditions
1,2,3,4-Tetrahydroquinolin-4-ol hydrochloride can be synthesized through several methods. One common method involves the reduction of quinoline derivatives. For instance, the reduction of 4-hydroxyquinoline using sodium borohydride in the presence of hydrochloric acid can yield this compound . Another method involves the cyclization of appropriate precursors under acidic conditions .
Industrial Production Methods
Industrial production of this compound typically involves large-scale reduction reactions using efficient catalysts and optimized reaction conditions to ensure high yield and purity. The use of continuous flow reactors and advanced purification techniques like crystallization and chromatography are common in industrial settings .
Chemical Reactions Analysis
Types of Reactions
1,2,3,4-Tetrahydroquinolin-4-ol hydrochloride undergoes various chemical reactions, including:
Oxidation: It can be oxidized to form quinoline derivatives.
Reduction: Further reduction can lead to the formation of fully saturated quinoline compounds.
Substitution: The hydroxyl group can be substituted with other functional groups under appropriate conditions.
Common Reagents and Conditions
Oxidation: Common oxidizing agents include potassium permanganate and chromium trioxide.
Reduction: Sodium borohydride and lithium aluminum hydride are frequently used reducing agents.
Substitution: Reagents like thionyl chloride and phosphorus tribromide are used for substitution reactions.
Major Products Formed
The major products formed from these reactions include various quinoline derivatives, fully saturated quinoline compounds, and substituted tetrahydroquinolines .
Scientific Research Applications
1,2,3,4-Tetrahydroquinolin-4-ol hydrochloride has several applications in scientific research:
Chemistry: It is used as a building block in the synthesis of complex organic molecules.
Biology: The compound is studied for its potential biological activities, including antimicrobial and anticancer properties.
Medicine: It serves as a precursor for the development of pharmaceutical agents targeting various diseases.
Industry: The compound is used in the production of dyes, pigments, and other industrial chemicals
Mechanism of Action
The mechanism of action of 1,2,3,4-tetrahydroquinolin-4-ol hydrochloride involves its interaction with specific molecular targets and pathways. It is known to inhibit certain enzymes and receptors, leading to its biological effects. The exact molecular targets and pathways can vary depending on the specific application and context of use .
Comparison with Similar Compounds
Similar Compounds
1,2,3,4-Tetrahydroisoquinoline: Another tetrahydroquinoline derivative with similar biological activities.
4-Hydroxyquinoline: A precursor in the synthesis of 1,2,3,4-tetrahydroquinolin-4-ol hydrochloride.
Quinoline: The parent compound from which many tetrahydroquinoline derivatives are synthesized
Uniqueness
This compound is unique due to its specific hydroxyl group at the 4-position, which imparts distinct chemical and biological properties. This functional group allows for various chemical modifications and enhances its potential as a versatile building block in synthetic chemistry .
Properties
Molecular Formula |
C9H12ClNO |
---|---|
Molecular Weight |
185.65 g/mol |
IUPAC Name |
1,2,3,4-tetrahydroquinolin-4-ol;hydrochloride |
InChI |
InChI=1S/C9H11NO.ClH/c11-9-5-6-10-8-4-2-1-3-7(8)9;/h1-4,9-11H,5-6H2;1H |
InChI Key |
AYZOELVHJSNQIZ-UHFFFAOYSA-N |
Canonical SMILES |
C1CNC2=CC=CC=C2C1O.Cl |
Origin of Product |
United States |
Disclaimer and Information on In-Vitro Research Products
Please be aware that all articles and product information presented on BenchChem are intended solely for informational purposes. The products available for purchase on BenchChem are specifically designed for in-vitro studies, which are conducted outside of living organisms. In-vitro studies, derived from the Latin term "in glass," involve experiments performed in controlled laboratory settings using cells or tissues. It is important to note that these products are not categorized as medicines or drugs, and they have not received approval from the FDA for the prevention, treatment, or cure of any medical condition, ailment, or disease. We must emphasize that any form of bodily introduction of these products into humans or animals is strictly prohibited by law. It is essential to adhere to these guidelines to ensure compliance with legal and ethical standards in research and experimentation.