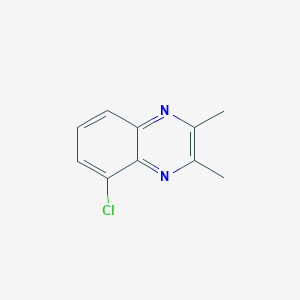
5-Chloro-2,3-dimethylquinoxaline
- Click on QUICK INQUIRY to receive a quote from our team of experts.
- With the quality product at a COMPETITIVE price, you can focus more on your research.
Overview
Description
5-Chloro-2,3-dimethylquinoxaline is a heterocyclic compound that belongs to the quinoxaline family. Quinoxalines are nitrogen-containing heterocycles known for their diverse biological activities and applications in various fields such as pharmaceuticals, agrochemicals, and materials science . The presence of chlorine and methyl groups in this compound enhances its chemical reactivity and potential for various applications.
Preparation Methods
Synthetic Routes and Reaction Conditions
5-Chloro-2,3-dimethylquinoxaline can be synthesized through several methods. One common approach involves the condensation of 2,3-diaminotoluene with 1,2-dichloroethane in the presence of a base such as potassium carbonate . The reaction is typically carried out under reflux conditions, and the product is purified through recrystallization.
Another method involves the cyclization of 2-chloro-3,4-dimethylbenzene-1,2-diamine with glyoxal in the presence of an acid catalyst . This reaction also requires reflux conditions and yields the desired quinoxaline derivative after purification.
Industrial Production Methods
Industrial production of this compound often employs continuous flow reactors to ensure consistent product quality and yield. The use of green chemistry principles, such as solvent-free conditions and recyclable catalysts, is also gaining popularity in industrial settings .
Chemical Reactions Analysis
Types of Reactions
5-Chloro-2,3-dimethylquinoxaline undergoes various chemical reactions, including:
Substitution: The chlorine atom in this compound can be substituted with other nucleophiles, such as amines or thiols, under appropriate conditions.
Common Reagents and Conditions
Oxidation: Hydrogen peroxide, m-chloroperbenzoic acid; typically carried out in an organic solvent like dichloromethane.
Reduction: Sodium borohydride, lithium aluminum hydride; reactions are usually performed in solvents like ethanol or tetrahydrofuran.
Major Products Formed
Oxidation: Quinoxaline N-oxides
Reduction: Dihydroquinoxalines
Substitution: Various substituted quinoxalines depending on the nucleophile used.
Scientific Research Applications
5-Chloro-2,3-dimethylquinoxaline has a wide range of applications in scientific research:
Mechanism of Action
The mechanism of action of 5-Chloro-2,3-dimethylquinoxaline varies depending on its application. In biological systems, it often targets specific enzymes or receptors. For example, as an acetylcholinesterase inhibitor, it binds to the enzyme’s active site, preventing the breakdown of acetylcholine and thereby enhancing cholinergic signaling . In anticancer applications, it may induce apoptosis in cancer cells by interacting with DNA or other cellular targets .
Comparison with Similar Compounds
Similar Compounds
2,3-Dimethylquinoxaline: Lacks the chlorine atom, resulting in different reactivity and biological activity.
5-Bromo-2,3-dimethylquinoxaline: Similar structure but with a bromine atom instead of chlorine, which can affect its chemical properties and applications.
5-Chloroquinoxaline: Lacks the methyl groups, leading to different steric and electronic effects.
Uniqueness
5-Chloro-2,3-dimethylquinoxaline is unique due to the presence of both chlorine and methyl groups, which enhance its reactivity and potential for diverse applications. The combination of these substituents allows for fine-tuning of its chemical and biological properties, making it a versatile compound in research and industry .
Properties
CAS No. |
17635-27-7 |
---|---|
Molecular Formula |
C10H9ClN2 |
Molecular Weight |
192.64 g/mol |
IUPAC Name |
5-chloro-2,3-dimethylquinoxaline |
InChI |
InChI=1S/C10H9ClN2/c1-6-7(2)13-10-8(11)4-3-5-9(10)12-6/h3-5H,1-2H3 |
InChI Key |
XACYYUSRWZUNSN-UHFFFAOYSA-N |
Canonical SMILES |
CC1=C(N=C2C(=N1)C=CC=C2Cl)C |
Origin of Product |
United States |
Disclaimer and Information on In-Vitro Research Products
Please be aware that all articles and product information presented on BenchChem are intended solely for informational purposes. The products available for purchase on BenchChem are specifically designed for in-vitro studies, which are conducted outside of living organisms. In-vitro studies, derived from the Latin term "in glass," involve experiments performed in controlled laboratory settings using cells or tissues. It is important to note that these products are not categorized as medicines or drugs, and they have not received approval from the FDA for the prevention, treatment, or cure of any medical condition, ailment, or disease. We must emphasize that any form of bodily introduction of these products into humans or animals is strictly prohibited by law. It is essential to adhere to these guidelines to ensure compliance with legal and ethical standards in research and experimentation.