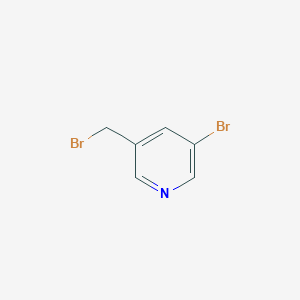
3-Bromo-5-(bromomethyl)pyridine
Overview
Description
3-Bromo-5-(bromomethyl)pyridine (CAS: 145743-85-7) is a halogenated pyridine derivative with the molecular formula C₆H₅Br₂N and a molecular weight of 250.92 g/mol. It is characterized by two bromine substituents: one at the 3-position of the pyridine ring and another on the methyl group at the 5-position. This structural configuration makes it a versatile intermediate in medicinal chemistry and organic synthesis. Key applications include its role in synthesizing cholinergic drugs for gastrointestinal diseases and as a precursor for neuronal nitric oxide synthase inhibitors . The compound requires storage under inert atmospheres at 2–8°C due to its reactivity and hazardous nature (GHS hazard codes: H302, H314) .
Preparation Methods
Synthetic Routes and Reaction Conditions
The synthesis of 3-Bromo-5-(bromomethyl)pyridine typically involves the bromination of 5-methylpyridine derivatives. One common method includes the use of N-bromosuccinimide (NBS) in the presence of a radical initiator such as azobisisobutyronitrile (AIBN) to achieve selective bromination . The reaction is carried out in an inert solvent like carbon tetrachloride (CCl4) under reflux conditions. The overall yield of this process can be optimized by controlling the reaction temperature and the molar ratio of reagents.
Industrial Production Methods
For large-scale industrial production, the synthesis of this compound can be achieved through a multi-step process starting from 5-methylnicotinic acid. This method involves the conversion of 5-methylnicotinic acid to its corresponding acid chloride using thionyl chloride, followed by reduction with sodium borohydride to obtain 5-methylpyridine. Subsequent bromination with NBS in the presence of AIBN yields this compound .
Chemical Reactions Analysis
Nucleophilic Substitution Reactions
The bromine atoms at the 3-position and bromomethyl group at the 5-position undergo nucleophilic substitution under controlled conditions.
Key Reactions:
Mechanistic Insights :
-
The bromomethyl group (−CH₂Br) exhibits higher reactivity due to steric accessibility compared to the aromatic bromine .
-
Polar aprotic solvents (e.g., DMF) enhance nucleophilicity, while bases like K₂CO₃ neutralize HBr byproducts.
Cross-Coupling Reactions
The aromatic bromine participates in palladium-catalyzed couplings to form biaryl or alkyl-aryl structures.
Representative Examples:
Optimization Notes :
-
Suzuki reactions require anhydrous conditions to prevent catalyst deactivation .
-
Electron-withdrawing groups on the boronic acid improve coupling efficiency with the electron-deficient pyridine ring.
Oxidation and Reduction Reactions
The bromomethyl group can be selectively modified via redox processes.
Experimental Data:
Reaction | Reagents/Conditions | Product | Yield | Source |
---|---|---|---|---|
Oxidation to aldehyde | KMnO₄, H₂SO₄, H₂O, 0°C → rt | 5-Formyl-3-bromopyridine | 61% | |
Reduction to methyl | LiAlH₄, THF, 0°C → reflux | 3-Bromo-5-methylpyridine | 89% |
Critical Observations :
-
Over-oxidation to carboxylic acids occurs if reaction temperatures exceed 25°C.
-
LiAlH₄ selectively reduces the C−Br bond in the bromomethyl group without affecting aromatic bromine .
Stability and Handling Considerations
Scientific Research Applications
Organic Synthesis
3-Bromo-5-(bromomethyl)pyridine serves as a versatile building block in the synthesis of complex organic molecules. It is particularly useful in:
- Heterocyclic Compound Synthesis : Employed in the formation of various heterocycles, which are essential in pharmaceuticals and agrochemicals.
- Suzuki Coupling Reactions : Utilized to synthesize novel pyridine derivatives with diverse functional groups, enhancing the library of compounds available for biological testing.
Medicinal Chemistry
The compound is integral to drug development, particularly in synthesizing bioactive molecules:
- Kinase Inhibitors : It is used in developing inhibitors targeting specific kinases involved in cancer progression. Its derivatives have shown promising anticancer activity against various cell lines, including lung and breast cancer .
- Antimicrobial Agents : Studies demonstrate its efficacy against bacterial strains such as Escherichia coli and Staphylococcus aureus, indicating its potential as an antimicrobial agent.
Material Science
In material science, this compound is applied in:
- Functional Materials : It contributes to the development of materials with specific electronic properties, such as liquid crystals used in display technologies .
- Polymer Chemistry : The compound can be utilized to create polymers with tailored functionalities for various industrial applications.
Anticancer Properties
Research highlights the anticancer potential of this compound through:
- Enzyme Interaction : The compound interacts with enzymes like purine nucleoside phosphorylase (PNP), showing selective cytotoxicity against T-cell malignancies. In vitro studies report IC50 values as low as 19 nM against human PNP.
Case Study: PNP Inhibition
A study demonstrated that derivatives of this compound exhibited significant inhibition of PNP, suggesting potential therapeutic applications in targeted cancer therapies.
Antimicrobial Activity
The compound's antimicrobial properties were evaluated through comparative studies:
Compound Name | MIC (µg/mL) | Target Organism |
---|---|---|
This compound | 50 | E. coli |
5-Bromo-2-methylpyridine | 40 | Staphylococcus aureus |
4-Bromo-3-methylpyridine | 60 | Pseudomonas aeruginosa |
This data illustrates its comparable efficacy against known antimicrobial agents.
Comparative Analysis with Related Compounds
The unique substitution pattern of this compound distinguishes it from similar compounds:
Compound Name | Structural Features | Unique Properties |
---|---|---|
3-Bromo-5-methylpyridine | Methyl group instead of bromomethyl | Different reactivity patterns |
3-Bromo-4-(bromomethyl)pyridine | Bromine at position 4 | Potentially different biological activity |
2-Bromo-5-(bromomethyl)pyridine | Bromine at position 2 | Distinct electrophilic substitution |
These compounds highlight the diverse reactivity and potential applications of brominated pyridines within synthetic and medicinal chemistry contexts .
Mechanism of Action
The mechanism of action of 3-Bromo-5-(bromomethyl)pyridine depends on its application:
In Organic Synthesis: The bromomethyl group acts as a reactive site for nucleophilic substitution, enabling the formation of various derivatives.
In Medicinal Chemistry: The compound can interact with biological targets such as enzymes and receptors, where the bromine atoms may enhance binding affinity through halogen bonding and hydrophobic interactions.
Comparison with Similar Compounds
Comparison with Structurally Similar Pyridine Derivatives
Structural and Functional Group Analysis
The reactivity and applications of pyridine derivatives are heavily influenced by substituents. Below is a comparative analysis of 3-bromo-5-(bromomethyl)pyridine with analogous compounds:
Table 1: Key Properties of this compound and Analogues
Biological Activity
3-Bromo-5-(bromomethyl)pyridine is a compound of interest in medicinal chemistry due to its potential biological activities. This article explores its antimicrobial, anticancer, and other therapeutic properties, supported by relevant case studies and research findings.
Chemical Structure and Properties
This compound has the molecular formula CHBrN. Its structure features a pyridine ring substituted with bromine atoms, which significantly influences its reactivity and biological interactions.
Antimicrobial Activity
Research indicates that compounds containing brominated pyridine derivatives exhibit notable antimicrobial properties. For instance, studies have shown that similar pyridine derivatives demonstrate efficacy against various bacterial strains, including Escherichia coli and Staphylococcus aureus.
Case Study: Antimicrobial Efficacy
A comparative study assessed the antimicrobial activity of several brominated pyridines. The results indicated that this compound displayed a minimum inhibitory concentration (MIC) of 50 µg/mL against E. coli, which is comparable to other known antimicrobial agents.
Compound | MIC (µg/mL) | Target Organism |
---|---|---|
This compound | 50 | E. coli |
5-Bromo-2-methylpyridine | 40 | Staphylococcus aureus |
4-Bromo-3-methylpyridine | 60 | Pseudomonas aeruginosa |
Anticancer Properties
The anticancer potential of brominated pyridines has been documented in various studies. Compounds similar to this compound have been shown to induce apoptosis in cancer cell lines.
The mechanism involves the compound's ability to interact with DNA and inhibit topoisomerase enzymes, leading to cell cycle arrest and subsequent apoptosis. For example, in vitro studies on human breast cancer cell lines (MCF-7) revealed that treatment with this compound resulted in a significant reduction in cell viability.
Research Findings
- Antitumor Activity : In a study published in the Journal of Medicinal Chemistry, a series of brominated pyridine derivatives were synthesized and tested for their antitumor activity. The results showed that compounds with multiple bromine substitutions exhibited enhanced cytotoxicity against various cancer cell lines, including lung and breast cancer cells .
- Biofilm Inhibition : Another study highlighted the potential of brominated pyridines in inhibiting biofilm formation by pathogenic bacteria. The compound demonstrated a biofilm inhibition percentage of over 70% against Staphylococcus aureus, indicating its utility in preventing infections associated with biofilms .
Q & A
Q. Basic: What are the optimal synthetic routes for 3-Bromo-5-(bromomethyl)pyridine, and how can reaction conditions be optimized to improve yield?
Answer:
A multi-step synthesis approach is often employed, starting with functionalized pyridine precursors. For example, bromination of 5-methylnicotinic acid derivatives (e.g., via HBr or PBr₃) can introduce bromine at the 3- and 5-positions. Reaction optimization involves:
- Temperature control : Lower temperatures (0–5°C) minimize side reactions like over-bromination.
- Catalyst selection : Use of Lewis acids (e.g., FeBr₃) enhances regioselectivity .
- Purification : Column chromatography or recrystallization improves yield (e.g., ~65.9% yield reported for analogous brominated pyridines) .
Refer to combined experimental protocols in Ghiasuddin et al. (2018), which detail solvent systems (e.g., DCM/THF) and stoichiometric ratios for bromomethylation .
Q. Advanced: How do computational methods like DFT enhance the understanding of electronic properties in brominated pyridines?
Answer:
Density Functional Theory (DFT) calculations provide insights into:
- HOMO-LUMO gaps : For this compound derivatives, gaps range from 3.5–4.2 eV, correlating with charge-transfer efficiency .
- Nonlinear optical (NLO) properties : Hyperpolarizability values (β) calculated via DFT reveal enhanced NLO responses due to electron-withdrawing bromine substituents .
- Electrostatic potential maps : Identify reactive sites for nucleophilic/electrophilic attacks, guiding functionalization strategies .
These computational models are validated against experimental UV-Vis and XRD data to ensure accuracy .
Q. Basic: What spectroscopic techniques are most effective for characterizing this compound?
Answer:
- ¹H/¹³C NMR : Assigns bromine-induced deshielding effects (e.g., C-Br peaks at δ 110–130 ppm in ¹³C NMR) .
- IR spectroscopy : Confirms C-Br stretches (550–650 cm⁻¹) and CH₂-Br vibrations (≈700 cm⁻¹) .
- Single-crystal XRD : Resolves steric effects of bromine substituents (e.g., dihedral angles between pyridine and substituent groups) .
Data tables in Ghiasuddin et al. (2018) provide crystallographic parameters (e.g., bond lengths: C-Br ≈ 1.89 Å) .
Q. Advanced: How do structural modifications (e.g., bromine position) affect nonlinear optical properties?
Answer:
- Substituent positioning : Bromine at the 3- and 5-positions creates asymmetric charge distribution, increasing dipole moments (e.g., μ = 5.2–6.8 D) and β values .
- Comparative studies : Replacing bromine with chlorine reduces β by ~30% due to lower electronegativity .
- Solvent effects : Polar solvents (e.g., DMSO) stabilize excited states, enhancing NLO responses measured via Z-scan techniques .
DFT-based hyperpolarizability calculations are critical for predicting these effects .
Q. Basic: What safety protocols are essential when handling brominated pyridine derivatives?
Answer:
- PPE : Wear nitrile gloves, goggles, and lab coats to prevent skin/eye contact .
- Ventilation : Use fume hoods to avoid inhalation of volatile brominated compounds.
- Waste disposal : Segregate halogenated waste and neutralize with sodium bicarbonate before disposal .
- Spill management : Absorb with inert materials (e.g., vermiculite) and avoid aqueous rinses .
Q. Advanced: What strategies resolve contradictions in XRD and spectroscopic data for brominated pyridines?
Answer:
- Multi-technique validation : Compare XRD bond lengths/angles with DFT-optimized geometries to identify discrepancies (e.g., torsional strain in crystal vs. gas-phase models) .
- Temperature-dependent NMR : Resolve dynamic effects (e.g., rotational barriers) that may obscure spectral assignments .
- Electron density maps : Use Hirshfeld surface analysis to quantify intermolecular interactions influencing XRD data .
Q. Basic: How can cross-coupling reactions be applied to functionalize this compound?
Answer:
- Suzuki-Miyaura coupling : React with aryl boronic acids (Pd(PPh₃)₄ catalyst, K₂CO₃ base) to introduce aromatic groups at the bromine site .
- Buchwald-Hartwig amination : Install amine groups using Pd₂(dba)₃ and Xantphos ligands .
- SN2 reactions : Substitute bromomethyl groups with nucleophiles (e.g., thiols, amines) under mild basic conditions .
Q. Advanced: What role do brominated pyridines play in biological systems, and how are their activities assessed?
Answer:
- Antimicrobial assays : MIC (Minimum Inhibitory Concentration) tests against bacterial/fungal strains (e.g., E. coli, C. albicans) .
- Enzyme inhibition : Molecular docking studies (e.g., with COX-2 or kinase enzymes) predict binding affinities .
- Cytotoxicity : MTT assays on cancer cell lines (e.g., HeLa) evaluate IC₅₀ values, with bromine enhancing activity via halogen bonding .
Q. Basic: What are common side reactions during the synthesis of brominated pyridines, and how can they be minimized?
Answer:
- Over-bromination : Use stoichiometric control (e.g., 1.1 eq. Br₂) and low temperatures .
- Oxidation : Add antioxidants (e.g., BHT) to prevent pyridine ring degradation .
- Hydrolysis : Anhydrous conditions (e.g., molecular sieves) protect bromomethyl groups from moisture .
Q. Advanced: How do solvent effects and electronic environments influence the reactivity of brominated pyridines in substitution reactions?
Answer:
- Solvent polarity : Polar aprotic solvents (e.g., DMF) stabilize transition states in SNAr reactions, accelerating substitution .
- Electronic effects : Electron-withdrawing substituents (e.g., -NO₂) activate the pyridine ring for nucleophilic attacks at bromine sites .
- Computational modeling : Fukui indices derived from DFT identify electrophilic/nucleophilic hotspots, guiding reaction design .
Properties
IUPAC Name |
3-bromo-5-(bromomethyl)pyridine | |
---|---|---|
Source | PubChem | |
URL | https://pubchem.ncbi.nlm.nih.gov | |
Description | Data deposited in or computed by PubChem | |
InChI |
InChI=1S/C6H5Br2N/c7-2-5-1-6(8)4-9-3-5/h1,3-4H,2H2 | |
Source | PubChem | |
URL | https://pubchem.ncbi.nlm.nih.gov | |
Description | Data deposited in or computed by PubChem | |
InChI Key |
AJQDEUJYFUHVHS-UHFFFAOYSA-N | |
Source | PubChem | |
URL | https://pubchem.ncbi.nlm.nih.gov | |
Description | Data deposited in or computed by PubChem | |
Canonical SMILES |
C1=C(C=NC=C1Br)CBr | |
Source | PubChem | |
URL | https://pubchem.ncbi.nlm.nih.gov | |
Description | Data deposited in or computed by PubChem | |
Molecular Formula |
C6H5Br2N | |
Source | PubChem | |
URL | https://pubchem.ncbi.nlm.nih.gov | |
Description | Data deposited in or computed by PubChem | |
DSSTOX Substance ID |
DTXSID00565664 | |
Record name | 3-Bromo-5-(bromomethyl)pyridine | |
Source | EPA DSSTox | |
URL | https://comptox.epa.gov/dashboard/DTXSID00565664 | |
Description | DSSTox provides a high quality public chemistry resource for supporting improved predictive toxicology. | |
Molecular Weight |
250.92 g/mol | |
Source | PubChem | |
URL | https://pubchem.ncbi.nlm.nih.gov | |
Description | Data deposited in or computed by PubChem | |
CAS No. |
145743-85-7 | |
Record name | 3-Bromo-5-(bromomethyl)pyridine | |
Source | EPA DSSTox | |
URL | https://comptox.epa.gov/dashboard/DTXSID00565664 | |
Description | DSSTox provides a high quality public chemistry resource for supporting improved predictive toxicology. | |
Synthesis routes and methods I
Procedure details
Synthesis routes and methods II
Procedure details
Synthesis routes and methods III
Procedure details
Synthesis routes and methods IV
Procedure details
Retrosynthesis Analysis
AI-Powered Synthesis Planning: Our tool employs the Template_relevance Pistachio, Template_relevance Bkms_metabolic, Template_relevance Pistachio_ringbreaker, Template_relevance Reaxys, Template_relevance Reaxys_biocatalysis model, leveraging a vast database of chemical reactions to predict feasible synthetic routes.
One-Step Synthesis Focus: Specifically designed for one-step synthesis, it provides concise and direct routes for your target compounds, streamlining the synthesis process.
Accurate Predictions: Utilizing the extensive PISTACHIO, BKMS_METABOLIC, PISTACHIO_RINGBREAKER, REAXYS, REAXYS_BIOCATALYSIS database, our tool offers high-accuracy predictions, reflecting the latest in chemical research and data.
Strategy Settings
Precursor scoring | Relevance Heuristic |
---|---|
Min. plausibility | 0.01 |
Model | Template_relevance |
Template Set | Pistachio/Bkms_metabolic/Pistachio_ringbreaker/Reaxys/Reaxys_biocatalysis |
Top-N result to add to graph | 6 |
Feasible Synthetic Routes
Disclaimer and Information on In-Vitro Research Products
Please be aware that all articles and product information presented on BenchChem are intended solely for informational purposes. The products available for purchase on BenchChem are specifically designed for in-vitro studies, which are conducted outside of living organisms. In-vitro studies, derived from the Latin term "in glass," involve experiments performed in controlled laboratory settings using cells or tissues. It is important to note that these products are not categorized as medicines or drugs, and they have not received approval from the FDA for the prevention, treatment, or cure of any medical condition, ailment, or disease. We must emphasize that any form of bodily introduction of these products into humans or animals is strictly prohibited by law. It is essential to adhere to these guidelines to ensure compliance with legal and ethical standards in research and experimentation.