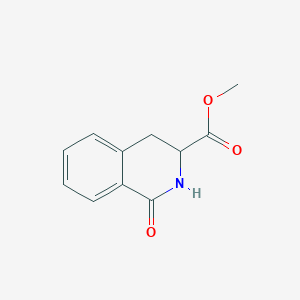
Methyl 1-oxo-1,2,3,4-tetrahydroisoquinoline-3-carboxylate
- Click on QUICK INQUIRY to receive a quote from our team of experts.
- With the quality product at a COMPETITIVE price, you can focus more on your research.
Overview
Description
Methyl 1-oxo-1,2,3,4-tetrahydroisoquinoline-3-carboxylate is a derivative of the tetrahydroisoquinoline family, which is known for its significant pharmacological potential. This compound is of particular interest due to its neuroprotective properties and its ability to interact with various biochemical pathways in the brain .
Preparation Methods
Synthetic Routes and Reaction Conditions
The synthesis of methyl 1-oxo-1,2,3,4-tetrahydroisoquinoline-3-carboxylate typically involves the Pictet-Spengler reaction, where phenylethylamine derivatives react with aldehydes or ketones in the presence of an acid catalyst . Another method involves the cyclization of an N-acyl derivative of β-phenylethylamine in the presence of dehydrating agents such as phosphorus oxychloride or zinc chloride .
Industrial Production Methods
Industrial production methods often utilize multicomponent reactions to improve yield and selectivity. These methods are designed to be environmentally friendly and economically viable, often employing transition metal-catalyzed cross-dehydrogenative coupling strategies .
Chemical Reactions Analysis
Types of Reactions
Methyl 1-oxo-1,2,3,4-tetrahydroisoquinoline-3-carboxylate undergoes various chemical reactions, including:
Reduction: Common reducing agents include sodium borohydride and lithium aluminum hydride.
Substitution: This compound can undergo nucleophilic substitution reactions, often facilitated by the presence of a leaving group.
Common Reagents and Conditions
Oxidation: Hydrogen peroxide, tert-butyl hydroperoxide.
Reduction: Sodium borohydride, lithium aluminum hydride.
Substitution: Various nucleophiles in the presence of a leaving group.
Major Products
The major products formed from these reactions depend on the specific conditions and reagents used. For example, oxidation can yield hydroxylated derivatives, while reduction can produce fully saturated compounds .
Scientific Research Applications
Methyl 1-oxo-1,2,3,4-tetrahydroisoquinoline-3-carboxylate has a wide range of scientific research applications:
Chemistry: Used as a building block for the synthesis of complex organic molecules.
Biology: Studied for its neuroprotective properties and its ability to modulate neurotransmitter levels.
Medicine: Investigated for its potential use in treating neurodegenerative diseases and as an antidepressant.
Industry: Utilized in the development of pharmaceuticals and agrochemicals.
Mechanism of Action
The compound exerts its effects primarily through the inhibition of monoamine oxidase (MAO) enzymes, which are involved in the catabolism of neurotransmitters such as dopamine and serotonin . By inhibiting these enzymes, the compound increases the levels of these neurotransmitters in the brain, leading to its neuroprotective and antidepressant effects . Additionally, it has been shown to scavenge free radicals and antagonize the glutamatergic system, further contributing to its neuroprotective properties .
Comparison with Similar Compounds
Similar Compounds
1-Methyl-1,2,3,4-tetrahydroisoquinoline: Known for its neuroprotective and antidepressant properties.
1,2,3,4-Tetrahydroisoquinoline: The simplest member of the tetrahydroisoquinoline family, with a broad spectrum of biological activities.
N-Benzyl-1,2,3,4-tetrahydroisoquinoline: Functions as an antineuroinflammatory agent.
Uniqueness
Methyl 1-oxo-1,2,3,4-tetrahydroisoquinoline-3-carboxylate stands out due to its specific combination of neuroprotective, antidepressant, and free radical scavenging properties. Its ability to modulate multiple biochemical pathways makes it a unique and valuable compound for scientific research and potential therapeutic applications .
Properties
IUPAC Name |
methyl 1-oxo-3,4-dihydro-2H-isoquinoline-3-carboxylate |
Source
|
---|---|---|
Details | Computed by LexiChem 2.6.6 (PubChem release 2019.06.18) | |
Source | PubChem | |
URL | https://pubchem.ncbi.nlm.nih.gov | |
Description | Data deposited in or computed by PubChem | |
InChI |
InChI=1S/C11H11NO3/c1-15-11(14)9-6-7-4-2-3-5-8(7)10(13)12-9/h2-5,9H,6H2,1H3,(H,12,13) |
Source
|
Details | Computed by InChI 1.0.5 (PubChem release 2019.06.18) | |
Source | PubChem | |
URL | https://pubchem.ncbi.nlm.nih.gov | |
Description | Data deposited in or computed by PubChem | |
InChI Key |
NRNCLSLTVLNNRL-UHFFFAOYSA-N |
Source
|
Details | Computed by InChI 1.0.5 (PubChem release 2019.06.18) | |
Source | PubChem | |
URL | https://pubchem.ncbi.nlm.nih.gov | |
Description | Data deposited in or computed by PubChem | |
Canonical SMILES |
COC(=O)C1CC2=CC=CC=C2C(=O)N1 |
Source
|
Details | Computed by OEChem 2.1.5 (PubChem release 2019.06.18) | |
Source | PubChem | |
URL | https://pubchem.ncbi.nlm.nih.gov | |
Description | Data deposited in or computed by PubChem | |
Molecular Formula |
C11H11NO3 |
Source
|
Details | Computed by PubChem 2.1 (PubChem release 2019.06.18) | |
Source | PubChem | |
URL | https://pubchem.ncbi.nlm.nih.gov | |
Description | Data deposited in or computed by PubChem | |
Molecular Weight |
205.21 g/mol |
Source
|
Details | Computed by PubChem 2.1 (PubChem release 2021.05.07) | |
Source | PubChem | |
URL | https://pubchem.ncbi.nlm.nih.gov | |
Description | Data deposited in or computed by PubChem | |
Disclaimer and Information on In-Vitro Research Products
Please be aware that all articles and product information presented on BenchChem are intended solely for informational purposes. The products available for purchase on BenchChem are specifically designed for in-vitro studies, which are conducted outside of living organisms. In-vitro studies, derived from the Latin term "in glass," involve experiments performed in controlled laboratory settings using cells or tissues. It is important to note that these products are not categorized as medicines or drugs, and they have not received approval from the FDA for the prevention, treatment, or cure of any medical condition, ailment, or disease. We must emphasize that any form of bodily introduction of these products into humans or animals is strictly prohibited by law. It is essential to adhere to these guidelines to ensure compliance with legal and ethical standards in research and experimentation.