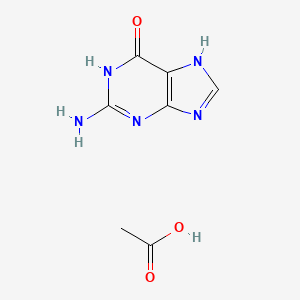
2-Amino-1H-purin-6(9H)-one acetate
- Click on QUICK INQUIRY to receive a quote from our team of experts.
- With the quality product at a COMPETITIVE price, you can focus more on your research.
Overview
Description
2-Amino-1H-purin-6(9H)-one acetate is a chemical compound with the molecular formula C5H5N5O·C2H4O2 It is a derivative of purine, a heterocyclic aromatic organic compound that plays a crucial role in biochemistry, particularly in the structure of DNA and RNA
Preparation Methods
Synthetic Routes and Reaction Conditions
The synthesis of 2-Amino-1H-purin-6(9H)-one acetate typically involves the reaction of 2-Amino-1H-purin-6(9H)-one with acetic acid. The reaction is carried out under controlled conditions to ensure the formation of the acetate salt. The general reaction scheme is as follows:
2-Amino-1H-purin-6(9H)-one+Acetic Acid→2-Amino-1H-purin-6(9H)-one acetate
Industrial Production Methods
In an industrial setting, the production of this compound may involve large-scale synthesis using automated reactors. The reaction conditions, such as temperature, pressure, and pH, are carefully monitored and controlled to optimize yield and purity. The product is then purified using techniques such as crystallization or chromatography.
Chemical Reactions Analysis
Types of Reactions
2-Amino-1H-purin-6(9H)-one acetate can undergo various chemical reactions, including:
Oxidation: The compound can be oxidized to form different derivatives.
Reduction: Reduction reactions can modify the functional groups attached to the purine ring.
Substitution: Nucleophilic substitution reactions can introduce new substituents onto the purine ring.
Common Reagents and Conditions
Oxidation: Common oxidizing agents include hydrogen peroxide and potassium permanganate.
Reduction: Reducing agents such as sodium borohydride or lithium aluminum hydride are used.
Substitution: Reagents like halogens or alkylating agents are employed under specific conditions to achieve substitution.
Major Products Formed
The major products formed from these reactions depend on the specific reagents and conditions used. For example, oxidation may yield hydroxylated derivatives, while substitution reactions can produce a variety of substituted purine compounds.
Scientific Research Applications
2-Amino-1H-purin-6(9H)-one acetate has several scientific research applications:
Chemistry: It is used as a building block in the synthesis of more complex molecules.
Biology: The compound is studied for its role in nucleic acid chemistry and its potential as a biochemical probe.
Medicine: Research is ongoing into its potential therapeutic applications, including antiviral and anticancer properties.
Industry: It is used in the development of pharmaceuticals and other chemical products.
Mechanism of Action
The mechanism of action of 2-Amino-1H-purin-6(9H)-one acetate involves its interaction with various molecular targets. In biological systems, it can interact with enzymes and nucleic acids, influencing biochemical pathways. The exact pathways and molecular targets depend on the specific application and context of use.
Comparison with Similar Compounds
Similar Compounds
2-Amino-1H-purin-6(9H)-one: The parent compound without the acetate group.
6-Mercaptopurine: A purine analog used in chemotherapy.
Adenine: A naturally occurring purine base found in DNA and RNA.
Uniqueness
2-Amino-1H-purin-6(9H)-one acetate is unique due to its specific chemical structure, which imparts distinct properties and reactivity compared to other purine derivatives. Its acetate group can influence its solubility, stability, and interaction with biological molecules, making it a valuable compound for various research and industrial applications.
Properties
Molecular Formula |
C7H9N5O3 |
---|---|
Molecular Weight |
211.18 g/mol |
IUPAC Name |
acetic acid;2-amino-1,7-dihydropurin-6-one |
InChI |
InChI=1S/C5H5N5O.C2H4O2/c6-5-9-3-2(4(11)10-5)7-1-8-3;1-2(3)4/h1H,(H4,6,7,8,9,10,11);1H3,(H,3,4) |
InChI Key |
KBYANRCVOIZOTD-UHFFFAOYSA-N |
Canonical SMILES |
CC(=O)O.C1=NC2=C(N1)C(=O)NC(=N2)N |
Origin of Product |
United States |
Disclaimer and Information on In-Vitro Research Products
Please be aware that all articles and product information presented on BenchChem are intended solely for informational purposes. The products available for purchase on BenchChem are specifically designed for in-vitro studies, which are conducted outside of living organisms. In-vitro studies, derived from the Latin term "in glass," involve experiments performed in controlled laboratory settings using cells or tissues. It is important to note that these products are not categorized as medicines or drugs, and they have not received approval from the FDA for the prevention, treatment, or cure of any medical condition, ailment, or disease. We must emphasize that any form of bodily introduction of these products into humans or animals is strictly prohibited by law. It is essential to adhere to these guidelines to ensure compliance with legal and ethical standards in research and experimentation.