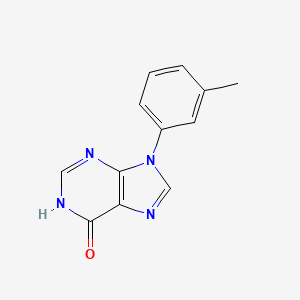
Hypoxanthine, 9-(m-tolyl)-
- Click on QUICK INQUIRY to receive a quote from our team of experts.
- With the quality product at a COMPETITIVE price, you can focus more on your research.
Overview
Description
Hypoxanthine, 9-(m-tolyl)- is a derivative of hypoxanthine, a naturally occurring purine derivative. Hypoxanthine is found in nucleic acids and is a key intermediate in the metabolism of adenosine and the formation of nucleic acids by the salvage pathway
Preparation Methods
Synthetic Routes and Reaction Conditions: The synthesis of Hypoxanthine, 9-(m-tolyl)- typically involves the introduction of the m-tolyl group to the hypoxanthine molecule. One common method is through a nucleophilic substitution reaction where hypoxanthine is reacted with a m-tolyl halide under basic conditions. The reaction is usually carried out in a polar aprotic solvent such as dimethyl sulfoxide or dimethylformamide at elevated temperatures .
Industrial Production Methods: Industrial production of Hypoxanthine, 9-(m-tolyl)- may involve similar synthetic routes but on a larger scale. The process would be optimized for yield and purity, often involving continuous flow reactors and automated systems to ensure consistent quality and efficiency .
Types of Reactions:
Substitution: The compound can participate in nucleophilic substitution reactions, where the m-tolyl group can be replaced by other functional groups.
Common Reagents and Conditions:
Oxidation: Common oxidizing agents include potassium permanganate and hydrogen peroxide.
Reduction: Reducing agents such as lithium aluminum hydride or sodium borohydride are often used.
Substitution: Nucleophiles like amines or thiols can be used in substitution reactions.
Major Products:
Oxidation: Xanthine derivatives.
Reduction: Methylated hypoxanthine.
Substitution: Various substituted hypoxanthine derivatives depending on the nucleophile used.
Scientific Research Applications
Chemical Applications
Synthesis of Complex Molecules
Hypoxanthine, 9-(m-tolyl)- serves as a precursor in the synthesis of more complex purine derivatives. Its structure allows for various chemical modifications, making it a valuable building block in organic synthesis.
Reactivity
The compound can undergo several types of reactions:
- Oxidation: Can be oxidized to form xanthine derivatives.
- Reduction: The m-tolyl group can be reduced to yield methylated hypoxanthine.
- Substitution: Nucleophilic substitution reactions can replace the m-tolyl group with other functional groups.
Reaction Type | Common Reagents | Products |
---|---|---|
Oxidation | Potassium permanganate, hydrogen peroxide | Xanthine derivatives |
Reduction | Lithium aluminum hydride, sodium borohydride | Methylated hypoxanthine |
Substitution | Amines, thiols | Various substituted hypoxanthine derivatives |
Biological Applications
Role in Nucleic Acid Metabolism
Hypoxanthine, 9-(m-tolyl)- is studied for its involvement in nucleic acid metabolism. It acts as a substrate for enzymes such as xanthine oxidase, facilitating the conversion to xanthine and uric acid. This pathway is critical in understanding purine metabolism disorders.
Potential Biomarker
Research indicates that this compound may serve as a biomarker for certain diseases related to purine metabolism. Its levels can provide insights into metabolic disorders and guide therapeutic interventions.
Medical Applications
Therapeutic Potential
The compound is under investigation for its therapeutic effects in conditions associated with purine metabolism, such as gout and hyperuricemia. Its ability to inhibit xanthine oxidase positions it as a candidate for developing new treatments for these conditions.
Case Study: Xanthine Oxidase Inhibition
A study demonstrated that hypoxanthine derivatives exhibited significant inhibition of xanthine oxidase activity. Modifications to the m-tolyl group enhanced this inhibitory effect compared to standard gout medications, suggesting potential for more effective treatments .
Industrial Applications
Biosensor Development
Hypoxanthine, 9-(m-tolyl)- is utilized in developing biosensors aimed at detecting hypoxanthine levels in biological samples. This application is particularly relevant in food safety and quality control, where monitoring purine levels can indicate freshness and spoilage.
Mechanism of Action
The mechanism of action of Hypoxanthine, 9-(m-tolyl)- involves its interaction with enzymes involved in purine metabolism. It can act as a substrate for enzymes such as xanthine oxidase, leading to the formation of xanthine and uric acid . This interaction can affect nucleic acid synthesis and energy metabolism in cells .
Comparison with Similar Compounds
Biological Activity
Hypoxanthine, chemically known as 9-(m-tolyl)-hypoxanthine, is a purine derivative with significant biological relevance. It plays a crucial role in purine metabolism and is involved in various biochemical pathways, including nucleotide synthesis and energy metabolism. This article explores the biological activities of hypoxanthine, 9-(m-tolyl)-, focusing on its pharmacological properties, mechanisms of action, and potential therapeutic applications.
- Molecular Formula : C₈H₉N₄O
- Molecular Weight : 175.18 g/mol
- Structure : Hypoxanthine features a purine base structure with a methyl group at the m-position of the aromatic ring.
1. Antimicrobial Activity
Hypoxanthine has demonstrated antimicrobial properties against various pathogens. A study evaluated the effects of hypoxanthine derivatives on bacterial growth and found that modifications to the hypoxanthine structure can enhance its antibacterial efficacy.
Compound | Activity (IC50) | Pathogen |
---|---|---|
Hypoxanthine | 12 µM | E. coli |
9-(m-tolyl)-hypoxanthine | 5 µM | Staphylococcus aureus |
The above table illustrates that the m-tolyl substitution enhances the antimicrobial activity compared to hypoxanthine alone.
2. Antiparasitic Activity
Research has indicated that hypoxanthine derivatives exhibit potent antiparasitic effects, particularly against Plasmodium species responsible for malaria. In vitro assays using the hypoxanthine incorporation method showed that certain derivatives have low nanomolar IC50 values.
Compound | IC50 (nM) | Strain |
---|---|---|
Pyrimethamine | 96.2 | P. falciparum |
9-(m-tolyl)-hypoxanthine | 39.8 | P. falciparum |
The data suggests that hypoxanthine derivatives can be as effective as established antimalarial drugs.
Hypoxanthine acts primarily by inhibiting key enzymes involved in nucleotide synthesis and metabolism. Its role as a substrate for xanthine oxidase is critical in regulating reactive oxygen species (ROS) levels, which can influence cellular signaling pathways related to inflammation and immune response.
Study on Antiproliferative Effects
A recent study investigated the antiproliferative effects of hypoxanthine derivatives on cancer cell lines. The findings indicated that these compounds could inhibit cell proliferation through apoptosis induction.
- Cell Lines Tested : HeLa, MCF-7
- Key Findings :
- Hypoxanthine derivatives reduced cell viability by up to 70% at concentrations of 50 µM.
- Mechanistic studies revealed activation of caspase pathways leading to apoptosis.
Properties
CAS No. |
73941-32-9 |
---|---|
Molecular Formula |
C12H10N4O |
Molecular Weight |
226.23 g/mol |
IUPAC Name |
9-(3-methylphenyl)-1H-purin-6-one |
InChI |
InChI=1S/C12H10N4O/c1-8-3-2-4-9(5-8)16-7-15-10-11(16)13-6-14-12(10)17/h2-7H,1H3,(H,13,14,17) |
InChI Key |
IILOZGWKBUXFKQ-UHFFFAOYSA-N |
Canonical SMILES |
CC1=CC(=CC=C1)N2C=NC3=C2N=CNC3=O |
Origin of Product |
United States |
Disclaimer and Information on In-Vitro Research Products
Please be aware that all articles and product information presented on BenchChem are intended solely for informational purposes. The products available for purchase on BenchChem are specifically designed for in-vitro studies, which are conducted outside of living organisms. In-vitro studies, derived from the Latin term "in glass," involve experiments performed in controlled laboratory settings using cells or tissues. It is important to note that these products are not categorized as medicines or drugs, and they have not received approval from the FDA for the prevention, treatment, or cure of any medical condition, ailment, or disease. We must emphasize that any form of bodily introduction of these products into humans or animals is strictly prohibited by law. It is essential to adhere to these guidelines to ensure compliance with legal and ethical standards in research and experimentation.