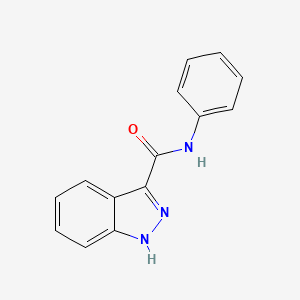
N-Phenyl-1H-indazole-3-carboxamide
- Click on QUICK INQUIRY to receive a quote from our team of experts.
- With the quality product at a COMPETITIVE price, you can focus more on your research.
Overview
Description
N-Phenyl-1H-indazole-3-carboxamide is a heterocyclic compound that belongs to the indazole family. Indazoles are known for their diverse biological activities and are used in various medicinal applications. The structure of this compound consists of an indazole ring fused with a phenyl group and a carboxamide functional group at the third position of the indazole ring.
Preparation Methods
Synthetic Routes and Reaction Conditions
The synthesis of N-Phenyl-1H-indazole-3-carboxamide can be achieved through several methods. One common approach involves the reaction of indazole with phenyl isocyanate under basic conditions. Another method includes the use of chlorocarbonyl compounds, where indazole reacts with chlorocarbonyl phenyl isocyanate in the presence of a base to form the desired product .
Industrial Production Methods
Industrial production of this compound typically involves large-scale synthesis using optimized reaction conditions to ensure high yield and purity. The use of transition metal catalysts, such as copper or palladium, can enhance the efficiency of the synthesis process .
Chemical Reactions Analysis
Types of Reactions
N-Phenyl-1H-indazole-3-carboxamide undergoes various chemical reactions, including:
Oxidation: The compound can be oxidized to form corresponding oxides.
Reduction: Reduction reactions can convert the carboxamide group to an amine.
Substitution: The phenyl group can undergo electrophilic or nucleophilic substitution reactions.
Common Reagents and Conditions
Oxidation: Common oxidizing agents include potassium permanganate and chromium trioxide.
Reduction: Reducing agents such as lithium aluminum hydride or sodium borohydride are used.
Substitution: Reagents like halogens, alkylating agents, and nucleophiles are employed under appropriate conditions.
Major Products
The major products formed from these reactions depend on the specific reagents and conditions used. For example, oxidation may yield indazole oxides, while reduction can produce indazole amines .
Scientific Research Applications
N-Phenyl-1H-indazole-3-carboxamide has a wide range of scientific research applications:
Chemistry: Used as a building block for the synthesis of more complex molecules.
Biology: Investigated for its potential as a bioactive compound with antimicrobial and anticancer properties.
Medicine: Explored as a potential therapeutic agent for various diseases, including cancer and inflammatory conditions.
Industry: Utilized in the development of new materials and chemical processes
Mechanism of Action
The mechanism of action of N-Phenyl-1H-indazole-3-carboxamide involves its interaction with specific molecular targets and pathways. It can act as an inhibitor of certain enzymes or receptors, leading to the modulation of biological processes. For example, it may inhibit protein kinases involved in cell signaling pathways, thereby affecting cell proliferation and survival .
Comparison with Similar Compounds
N-Phenyl-1H-indazole-3-carboxamide can be compared with other indazole derivatives, such as:
- N-Phenyl-1H-indazole-3-carboxylate
- This compound derivatives with different substituents
- Indazole-3-carboxamides with various functional groups
These compounds share similar core structures but differ in their functional groups, leading to variations in their chemical properties and biological activities.
Properties
CAS No. |
23706-99-2 |
---|---|
Molecular Formula |
C14H11N3O |
Molecular Weight |
237.26 g/mol |
IUPAC Name |
N-phenyl-1H-indazole-3-carboxamide |
InChI |
InChI=1S/C14H11N3O/c18-14(15-10-6-2-1-3-7-10)13-11-8-4-5-9-12(11)16-17-13/h1-9H,(H,15,18)(H,16,17) |
InChI Key |
XQJXNILPLUCHQG-UHFFFAOYSA-N |
Canonical SMILES |
C1=CC=C(C=C1)NC(=O)C2=NNC3=CC=CC=C32 |
Origin of Product |
United States |
Disclaimer and Information on In-Vitro Research Products
Please be aware that all articles and product information presented on BenchChem are intended solely for informational purposes. The products available for purchase on BenchChem are specifically designed for in-vitro studies, which are conducted outside of living organisms. In-vitro studies, derived from the Latin term "in glass," involve experiments performed in controlled laboratory settings using cells or tissues. It is important to note that these products are not categorized as medicines or drugs, and they have not received approval from the FDA for the prevention, treatment, or cure of any medical condition, ailment, or disease. We must emphasize that any form of bodily introduction of these products into humans or animals is strictly prohibited by law. It is essential to adhere to these guidelines to ensure compliance with legal and ethical standards in research and experimentation.