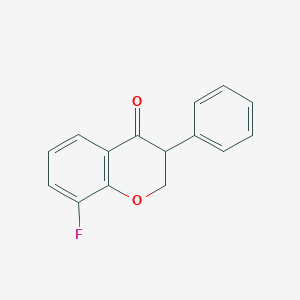
8-Fluoro-3-phenylchroman-4-one
- Click on QUICK INQUIRY to receive a quote from our team of experts.
- With the quality product at a COMPETITIVE price, you can focus more on your research.
Overview
Description
8-Fluoro-3-phenylchroman-4-one is a synthetic organic compound belonging to the chromanone family. Chromanones are heterocyclic compounds characterized by a benzene ring fused to a dihydropyranone ring. The presence of a fluorine atom at the 8th position and a phenyl group at the 3rd position distinguishes this compound from other chromanone derivatives.
Preparation Methods
Synthetic Routes and Reaction Conditions: The synthesis of 8-Fluoro-3-phenylchroman-4-one typically involves the annulation of a fluorinated benzene derivative with a suitable chromanone precursor. One common method is the gold(I)-catalyzed annulation reaction, which allows for the efficient formation of the chromanone ring system . The reaction conditions often include the use of gold(I) catalysts, such as gold(I) chloride, in the presence of a base like potassium carbonate, and solvents like dichloromethane or toluene.
Industrial Production Methods: Industrial production of this compound may involve similar synthetic routes but on a larger scale. Optimization of reaction conditions, such as temperature, pressure, and catalyst concentration, is crucial to achieve high yields and purity. Continuous flow reactors and automated synthesis platforms can enhance the efficiency and scalability of the production process .
Chemical Reactions Analysis
Types of Reactions: 8-Fluoro-3-phenylchroman-4-one undergoes various chemical reactions, including:
Oxidation: The compound can be oxidized to form corresponding quinones or other oxidized derivatives.
Reduction: Reduction reactions can convert the chromanone ring to a chromanol ring.
Substitution: The fluorine atom and phenyl group can participate in nucleophilic or electrophilic substitution reactions.
Common Reagents and Conditions:
Oxidation: Common oxidizing agents include potassium permanganate, chromium trioxide, and hydrogen peroxide.
Reduction: Reducing agents such as sodium borohydride or lithium aluminum hydride are often used.
Substitution: Reagents like halogens, alkylating agents, and nucleophiles can be employed under appropriate conditions.
Major Products: The major products formed from these reactions depend on the specific reagents and conditions used. For example, oxidation may yield quinones, while reduction can produce chromanols .
Scientific Research Applications
Mechanism of Action
The mechanism of action of 8-Fluoro-3-phenylchroman-4-one involves its interaction with specific molecular targets and pathways. For instance, as an aromatase inhibitor, it binds to the active site of the enzyme, preventing the conversion of androgens to estrogens. This inhibition can reduce estrogen levels, which is beneficial in treating estrogen-dependent cancers such as breast cancer . The compound may also interact with other molecular targets, such as kinases and receptors, modulating various cellular pathways .
Comparison with Similar Compounds
3-Phenylchroman-4-one: Lacks the fluorine atom at the 8th position, resulting in different chemical and biological properties.
6-Fluoro-3-phenylchroman-4-one: Fluorine atom is at the 6th position instead of the 8th, leading to variations in reactivity and potency.
6-Methoxy-3-phenylchroman-4-one: Contains a methoxy group instead of a fluorine atom, affecting its pharmacological profile.
Uniqueness: 8-Fluoro-3-phenylchroman-4-one’s unique structure, with the fluorine atom at the 8th position, imparts distinct chemical reactivity and biological activity. This uniqueness makes it a valuable compound for developing targeted therapies and studying structure-activity relationships .
Biological Activity
8-Fluoro-3-phenylchroman-4-one, a compound belonging to the isoflavanone class, has garnered attention due to its potential biological activities, particularly as an aromatase inhibitor. Aromatase plays a crucial role in estrogen biosynthesis, making it a significant target for therapeutic interventions in hormone-dependent cancers, especially breast cancer.
The synthesis of this compound involves a one-step gold(I)-catalyzed annulation reaction using 3-fluorosalicylaldehyde and phenylacetylene. This method allows for the efficient production of fluorinated isoflavanones, which are characterized by their unique structural features that enhance their biological activities .
Aromatase Inhibition
In vitro studies have demonstrated that this compound exhibits significant inhibitory activity against aromatase. The compound's inhibitory potency was assessed using fluorescence-based enzymatic assays with recombinant human aromatase. The results indicated that this compound and its derivatives possess IC₅₀ values ranging from 0.8 μM to 43 μM, indicating varying degrees of efficacy .
Table 1: Aromatase Inhibition Potency of Isoflavanone Derivatives
Compound | IC₅₀ (μM) |
---|---|
6-Methoxy-3-(pyridin-3-yl)chroman-4-one | 2.5 |
6-Fluoro-3-(pyridin-3-yl)chroman-4-one | 0.8 |
This compound | 43 |
Anti-Proliferative Effects
The anti-proliferative effects of this compound were evaluated on the MCF-7 breast cancer cell line. Although some derivatives showed promising results, the specific compound was noted to have limited activity in cell assays, suggesting that structural modifications could enhance its efficacy .
Case Study: MCF-7 Cell Line Analysis
In a controlled study, MCF-7 cells were treated with varying concentrations of this compound. The outcomes revealed that while the compound inhibited cell proliferation at higher concentrations, its effectiveness was significantly lower compared to established aromatase inhibitors like letrozole (IC₅₀ = ~20 nM) .
The mechanism underlying the biological activity of this compound involves hydrophobic interactions and hydrogen bonding with the aromatase enzyme. Molecular docking simulations have provided insights into how these interactions facilitate the inhibition of estrogen biosynthesis .
Figure 1: Interaction Model of Aromatase and Isoflavanone Derivatives
Interaction Model (Note: Image link is illustrative)
Toxicity and Safety Profile
Preliminary assessments indicate that fluorinated isoflavanones, including this compound, exhibit low to medium predicted toxicity profiles. These compounds are generally considered to have low mutagenic and tumorigenic potential, which is critical for their development as therapeutic agents .
Properties
Molecular Formula |
C15H11FO2 |
---|---|
Molecular Weight |
242.24 g/mol |
IUPAC Name |
8-fluoro-3-phenyl-2,3-dihydrochromen-4-one |
InChI |
InChI=1S/C15H11FO2/c16-13-8-4-7-11-14(17)12(9-18-15(11)13)10-5-2-1-3-6-10/h1-8,12H,9H2 |
InChI Key |
SFLDITPKEYNRDS-UHFFFAOYSA-N |
Canonical SMILES |
C1C(C(=O)C2=C(O1)C(=CC=C2)F)C3=CC=CC=C3 |
Origin of Product |
United States |
Disclaimer and Information on In-Vitro Research Products
Please be aware that all articles and product information presented on BenchChem are intended solely for informational purposes. The products available for purchase on BenchChem are specifically designed for in-vitro studies, which are conducted outside of living organisms. In-vitro studies, derived from the Latin term "in glass," involve experiments performed in controlled laboratory settings using cells or tissues. It is important to note that these products are not categorized as medicines or drugs, and they have not received approval from the FDA for the prevention, treatment, or cure of any medical condition, ailment, or disease. We must emphasize that any form of bodily introduction of these products into humans or animals is strictly prohibited by law. It is essential to adhere to these guidelines to ensure compliance with legal and ethical standards in research and experimentation.