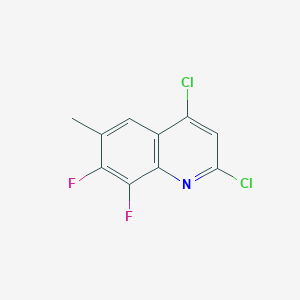
2,4-Dichloro-7,8-difluoro-6-methylquinoline
- Click on QUICK INQUIRY to receive a quote from our team of experts.
- With the quality product at a COMPETITIVE price, you can focus more on your research.
Overview
Description
2,4-Dichloro-7,8-difluoro-6-methylquinoline is a fluorinated quinoline derivative with the molecular formula C10H5Cl2F2N and a molecular weight of 248.06 g/mol . This compound is part of a broader class of fluorinated quinolines, which are known for their diverse applications in medicinal chemistry, agriculture, and material science .
Preparation Methods
Synthetic Routes and Reaction Conditions: The synthesis of 2,4-Dichloro-7,8-difluoro-6-methylquinoline typically involves the cyclization and cycloaddition reactions, displacements of halogen atoms, and direct fluorinations . One common method includes the reaction of 2,4-dihydroxy-6-fluoroquinoline with phosphorus oxychloride in acetonitrile at elevated temperatures (65-85°C) followed by purification through column chromatography .
Industrial Production Methods: Industrial production methods for this compound often involve large-scale cyclization reactions under controlled conditions to ensure high yield and purity. The use of organometallic compounds and cross-coupling reactions are also prevalent in industrial settings to achieve efficient synthesis .
Chemical Reactions Analysis
Types of Reactions: 2,4-Dichloro-7,8-difluoro-6-methylquinoline undergoes various chemical reactions, including:
Nucleophilic Substitution: Displacement of chlorine or fluorine atoms.
Cross-Coupling Reactions: Such as Suzuki-Miyaura coupling, which involves the formation of carbon-carbon bonds.
Common Reagents and Conditions:
Nucleophilic Substitution: Typically involves nucleophiles like amines or thiols under basic conditions.
Cross-Coupling Reactions: Utilizes palladium catalysts and boron reagents under mild conditions.
Major Products: The major products formed from these reactions include substituted quinolines with potential biological activities .
Scientific Research Applications
2,4-Dichloro-7,8-difluoro-6-methylquinoline has a wide range of applications in scientific research:
Chemistry: Used as a building block for the synthesis of more complex molecules.
Biology: Investigated for its potential as an enzyme inhibitor and antibacterial agent.
Medicine: Explored for its antineoplastic and antiviral properties.
Industry: Utilized in the development of liquid crystals and cyanine dyes.
Mechanism of Action
The mechanism of action of 2,4-Dichloro-7,8-difluoro-6-methylquinoline involves its interaction with specific molecular targets, such as enzymes. The incorporation of fluorine atoms enhances its biological activity by increasing its binding affinity to target proteins and improving its metabolic stability . The pathways involved often include inhibition of enzyme activity, leading to antibacterial or antineoplastic effects .
Comparison with Similar Compounds
- 2,4-Dichloro-6-fluoroquinoline
- 2,4-Dichloro-6,7-dimethoxyquinazoline
- 7-bromo-2,4-dichloro-6,8-difluoro-3-methylquinoline
Uniqueness: 2,4-Dichloro-7,8-difluoro-6-methylquinoline stands out due to its unique combination of chlorine and fluorine substitutions, which confer enhanced biological activity and stability compared to other quinoline derivatives .
Biological Activity
2,4-Dichloro-7,8-difluoro-6-methylquinoline is a member of the quinoline family, which has garnered attention due to its diverse biological activities. This compound is particularly noted for its potential applications in pharmacology, including antibacterial and anticancer properties. This article delves into the biological activity of this compound, summarizing key research findings, case studies, and relevant data.
Chemical Structure and Properties
The chemical structure of this compound can be represented as follows:
- Molecular Formula : C10H6Cl2F2N
- Molecular Weight : 253.06 g/mol
This compound features two chlorine atoms and two fluorine atoms substituted on the quinoline ring, which contributes to its unique chemical reactivity and biological activity.
Antibacterial Properties
Research indicates that this compound exhibits significant antibacterial activity. A study demonstrated that derivatives of quinoline compounds showed varying degrees of effectiveness against Gram-positive and Gram-negative bacteria. Specifically, compounds with similar structures exhibited minimum inhibitory concentrations (MICs) ranging from 1×10−4 to 1×10−6 mg/mL against pathogens such as Staphylococcus aureus and Escherichia coli .
Table 1: Antibacterial Activity of Quinoline Derivatives
Compound | MIC (mg/mL) | Target Bacteria |
---|---|---|
This compound | 1×10−5 | S. aureus |
Other Quinoline Derivative | 1×10−6 | E. coli |
Standard Drug | 1×10−4 | Ciprofloxacin |
Anticancer Activity
In vitro studies have shown that this compound can inhibit the growth of various cancer cell lines. A notable investigation assessed its cytotoxicity against HeLa (cervical cancer), A549 (lung cancer), and MCF-7 (breast cancer) cells. The results indicated that the compound exhibited IC50 values in the range of 5–19 µM, demonstrating a higher potency compared to traditional chemotherapeutics like cisplatin .
Table 2: Cytotoxicity Against Cancer Cell Lines
Cell Line | IC50 (µM) | Comparison Drug | IC50 (µM) |
---|---|---|---|
HeLa | 10 | Cisplatin | 21 |
MCF-7 | 7 | Cisplatin | 27 |
A549 | 15 | Cisplatin | 30 |
The mechanism by which this compound exerts its biological effects may involve interaction with DNA and inhibition of critical cellular processes. Studies suggest that quinoline derivatives can intercalate into DNA or inhibit topoisomerases, leading to disruption in replication and transcription processes .
Case Study 1: Antibacterial Efficacy
In a comparative study on the antibacterial efficacy of various quinoline derivatives, it was found that those with fluorinated substitutions displayed enhanced activity against resistant strains of bacteria. The study highlighted that the introduction of fluorine increased lipophilicity and improved membrane permeability, thus enhancing antibacterial action .
Case Study 2: Anticancer Potential
A study focused on the anticancer potential of various quinoline derivatives indicated that those with halogen substitutions demonstrated significant cytotoxicity against a range of cancer cell lines. The findings suggested that these modifications could lead to the development of novel anticancer agents with improved selectivity for cancer cells over normal cells .
Properties
Molecular Formula |
C10H5Cl2F2N |
---|---|
Molecular Weight |
248.05 g/mol |
IUPAC Name |
2,4-dichloro-7,8-difluoro-6-methylquinoline |
InChI |
InChI=1S/C10H5Cl2F2N/c1-4-2-5-6(11)3-7(12)15-10(5)9(14)8(4)13/h2-3H,1H3 |
InChI Key |
CMXMCSAKGRJSJF-UHFFFAOYSA-N |
Canonical SMILES |
CC1=CC2=C(C(=C1F)F)N=C(C=C2Cl)Cl |
Origin of Product |
United States |
Disclaimer and Information on In-Vitro Research Products
Please be aware that all articles and product information presented on BenchChem are intended solely for informational purposes. The products available for purchase on BenchChem are specifically designed for in-vitro studies, which are conducted outside of living organisms. In-vitro studies, derived from the Latin term "in glass," involve experiments performed in controlled laboratory settings using cells or tissues. It is important to note that these products are not categorized as medicines or drugs, and they have not received approval from the FDA for the prevention, treatment, or cure of any medical condition, ailment, or disease. We must emphasize that any form of bodily introduction of these products into humans or animals is strictly prohibited by law. It is essential to adhere to these guidelines to ensure compliance with legal and ethical standards in research and experimentation.