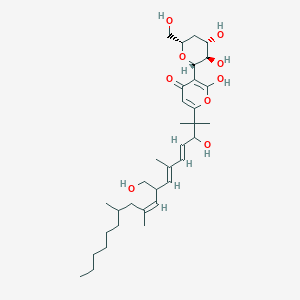
Fusapyrone
Overview
Description
Mechanism of Action
Target of Action
Fusapyrone, also known as Neothis compound, is a secondary metabolite produced by the phytopathogenic fungus Fusarium mangiferae . The primary target of this compound is the polyketide synthase (PKS) FmPKS40 . This enzyme plays a crucial role in the biosynthesis of this compound .
Mode of Action
This compound interacts with its target, the polyketide synthase FmPKS40, to facilitate its own biosynthesis . The interaction between this compound and FmPKS40 is essential for the production of this compound .
Biochemical Pathways
This compound biosynthesis is facilitated by a seven-gene cluster . The key enzyme involved in this compound biosynthesis is FmPKS40 . The loss of FmKMT1, a H3K9 methyltransferase, resulted in an almost complete loss of this compound . This suggests that the biosynthesis of this compound is regulated by posttranslational modifications of histones .
Pharmacokinetics
The bioavailability of this compound is likely to be influenced by its interaction with the polyketide synthase fmpks40 .
Result of Action
The molecular and cellular effects of this compound’s action are primarily related to its role in the biosynthesis of this compound . The loss of FmKMT1, a H3K9 methyltransferase, resulted in an almost complete loss of this compound , indicating that this compound plays a significant role in the regulation of fungal secondary metabolite biosynthesis .
Action Environment
This suggests that environmental factors such as nitrogen availability can influence the action, efficacy, and stability of this compound .
Biochemical Analysis
Biochemical Properties
Fusapyrone interacts with various biomolecules in biochemical reactions. It is synthesized by a diverse range of synthetic methods, resulting in the formation of various derivatives through chemical modifications . The polyketide synthase (PKS) FmPKS40 is involved in this compound biosynthesis . The C-2, C-4, and C-6 positions of the 2-pyrone are electrophilic and follow the nucleophilic attack .
Cellular Effects
This compound has significant effects on various types of cells and cellular processes. During plant infection, Fusarium mangiferae, which produces this compound, leads to severe adverse defects on plant health
Molecular Mechanism
The molecular mechanism of this compound involves its interactions with biomolecules at the molecular level. The polyketide synthase FmPKS40 is involved in this compound biosynthesis
Preparation Methods
Synthetic Routes and Reaction Conditions: Fusapyrone is primarily biosynthesized by the fungus Fusarium mangiferae. The biosynthesis involves the polyketide synthase enzyme FmPKS40, which is crucial for the production of this compound . The process is regulated by the H3K9 methyltransferase enzyme FmKmt1, which affects the chromatin structure and, consequently, the production of secondary metabolites .
Industrial Production Methods: Industrial production of this compound is typically achieved through fermentation processes involving Fusarium species. The fermentation conditions, including temperature, pH, and nutrient availability, are optimized to maximize the yield of this compound . The compound is then extracted and purified using standard chromatographic techniques.
Chemical Reactions Analysis
Types of Reactions: Fusapyrone undergoes various chemical reactions, including:
Oxidation: this compound can be oxidized to form various oxidized derivatives.
Reduction: Reduction reactions can modify the functional groups present in this compound.
Substitution: Substitution reactions can introduce different functional groups into the this compound molecule.
Common Reagents and Conditions:
Oxidation: Common oxidizing agents include potassium permanganate and hydrogen peroxide.
Reduction: Reducing agents such as sodium borohydride and lithium aluminum hydride are used.
Substitution: Reagents like halogens and nucleophiles are employed under various conditions.
Major Products: The major products formed from these reactions include various oxidized, reduced, and substituted derivatives of this compound, which can have different biological activities and properties .
Scientific Research Applications
Fusapyrone has several scientific research applications, including:
Comparison with Similar Compounds
Fusapyrone can be compared with other similar compounds, such as:
Deoxythis compound: Another γ-pyrone produced by Fusarium species, which also exhibits antifungal properties.
Fusintespyrone A: A glycoside isolated from Fusarium species, showing antifungal activity.
Cerevisterolside A: Another glycoside with a similar structure and antifungal properties.
Uniqueness: this compound’s unique structure, particularly the presence of the 4-deoxy-β-xylo-hexopyranosyl C-glycosyl moiety, distinguishes it from other similar compounds.
Properties
IUPAC Name |
3-[(2S,3R,4S,6S)-3,4-dihydroxy-6-(hydroxymethyl)oxan-2-yl]-4-hydroxy-6-[(4E,6E,9Z)-3-hydroxy-8-(hydroxymethyl)-2,6,10,12-tetramethyloctadeca-4,6,9-trien-2-yl]pyran-2-one | |
---|---|---|
Source | PubChem | |
URL | https://pubchem.ncbi.nlm.nih.gov | |
Description | Data deposited in or computed by PubChem | |
InChI |
InChI=1S/C34H54O9/c1-7-8-9-10-11-21(2)14-23(4)16-24(19-35)15-22(3)12-13-28(39)34(5,6)29-18-26(37)30(33(41)43-29)32-31(40)27(38)17-25(20-36)42-32/h12-13,15-16,18,21,24-25,27-28,31-32,35-40H,7-11,14,17,19-20H2,1-6H3/b13-12+,22-15+,23-16-/t21?,24?,25-,27-,28?,31+,32-/m0/s1 | |
Source | PubChem | |
URL | https://pubchem.ncbi.nlm.nih.gov | |
Description | Data deposited in or computed by PubChem | |
InChI Key |
HEECQDWUNPZALD-ZBNIOBMFSA-N | |
Source | PubChem | |
URL | https://pubchem.ncbi.nlm.nih.gov | |
Description | Data deposited in or computed by PubChem | |
Canonical SMILES |
CCCCCCC(C)CC(=CC(CO)C=C(C)C=CC(C(C)(C)C1=CC(=C(C(=O)O1)C2C(C(CC(O2)CO)O)O)O)O)C | |
Source | PubChem | |
URL | https://pubchem.ncbi.nlm.nih.gov | |
Description | Data deposited in or computed by PubChem | |
Isomeric SMILES |
CCCCCCC(C)C/C(=C\C(CO)/C=C(\C)/C=C/C(C(C)(C)C1=CC(=C(C(=O)O1)[C@H]2[C@@H]([C@H](C[C@H](O2)CO)O)O)O)O)/C | |
Source | PubChem | |
URL | https://pubchem.ncbi.nlm.nih.gov | |
Description | Data deposited in or computed by PubChem | |
Molecular Formula |
C34H54O9 | |
Source | PubChem | |
URL | https://pubchem.ncbi.nlm.nih.gov | |
Description | Data deposited in or computed by PubChem | |
Molecular Weight |
606.8 g/mol | |
Source | PubChem | |
URL | https://pubchem.ncbi.nlm.nih.gov | |
Description | Data deposited in or computed by PubChem | |
Retrosynthesis Analysis
AI-Powered Synthesis Planning: Our tool employs the Template_relevance Pistachio, Template_relevance Bkms_metabolic, Template_relevance Pistachio_ringbreaker, Template_relevance Reaxys, Template_relevance Reaxys_biocatalysis model, leveraging a vast database of chemical reactions to predict feasible synthetic routes.
One-Step Synthesis Focus: Specifically designed for one-step synthesis, it provides concise and direct routes for your target compounds, streamlining the synthesis process.
Accurate Predictions: Utilizing the extensive PISTACHIO, BKMS_METABOLIC, PISTACHIO_RINGBREAKER, REAXYS, REAXYS_BIOCATALYSIS database, our tool offers high-accuracy predictions, reflecting the latest in chemical research and data.
Strategy Settings
Precursor scoring | Relevance Heuristic |
---|---|
Min. plausibility | 0.01 |
Model | Template_relevance |
Template Set | Pistachio/Bkms_metabolic/Pistachio_ringbreaker/Reaxys/Reaxys_biocatalysis |
Top-N result to add to graph | 6 |
Feasible Synthetic Routes
Disclaimer and Information on In-Vitro Research Products
Please be aware that all articles and product information presented on BenchChem are intended solely for informational purposes. The products available for purchase on BenchChem are specifically designed for in-vitro studies, which are conducted outside of living organisms. In-vitro studies, derived from the Latin term "in glass," involve experiments performed in controlled laboratory settings using cells or tissues. It is important to note that these products are not categorized as medicines or drugs, and they have not received approval from the FDA for the prevention, treatment, or cure of any medical condition, ailment, or disease. We must emphasize that any form of bodily introduction of these products into humans or animals is strictly prohibited by law. It is essential to adhere to these guidelines to ensure compliance with legal and ethical standards in research and experimentation.