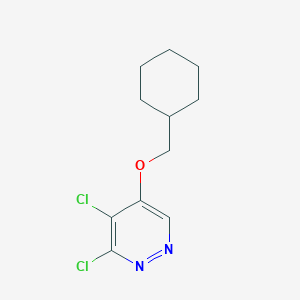
3,4-Dichloro-5-(cyclohexylmethoxy)pyridazine
- Click on QUICK INQUIRY to receive a quote from our team of experts.
- With the quality product at a COMPETITIVE price, you can focus more on your research.
Overview
Description
3,4-Dichloro-5-(cyclohexylmethoxy)pyridazine is a chemical compound with the molecular formula C11H14Cl2N2O. It is a member of the pyridazine family, which is characterized by a six-membered ring containing two adjacent nitrogen atoms.
Preparation Methods
The synthesis of 3,4-Dichloro-5-(cyclohexylmethoxy)pyridazine typically involves the reaction of 3,4-dichloropyridazine with cyclohexylmethanol under specific conditions. The reaction is usually carried out in the presence of a base, such as potassium carbonate, and a solvent, such as dimethylformamide (DMF). The reaction mixture is heated to facilitate the formation of the desired product .
Industrial production methods for this compound may involve similar synthetic routes but are optimized for large-scale production. This includes the use of continuous flow reactors and automated systems to ensure consistent quality and yield .
Chemical Reactions Analysis
3,4-Dichloro-5-(cyclohexylmethoxy)pyridazine undergoes various chemical reactions, including:
Substitution Reactions: The chlorine atoms in the compound can be substituted with other nucleophiles, such as amines or thiols, under appropriate conditions.
Oxidation Reactions: The compound can be oxidized to form various oxidized derivatives, depending on the reagents and conditions used.
Reduction Reactions: Reduction of the compound can lead to the formation of reduced derivatives, which may have different chemical and physical properties.
Common reagents used in these reactions include sodium hydride, lithium aluminum hydride, and various oxidizing agents such as potassium permanganate. The major products formed from these reactions depend on the specific reagents and conditions employed .
Scientific Research Applications
3,4-Dichloro-5-(cyclohexylmethoxy)pyridazine has several scientific research applications:
Chemistry: It is used as an intermediate in the synthesis of more complex chemical compounds.
Biology: The compound is studied for its potential biological activities, including antimicrobial and anticancer properties.
Medicine: Due to its potential biological activities, the compound is explored for its use in drug development.
Industry: The compound is used in the production of agrochemicals and other industrial products.
Mechanism of Action
The mechanism of action of 3,4-Dichloro-5-(cyclohexylmethoxy)pyridazine involves its interaction with specific molecular targets in biological systems. The compound may inhibit certain enzymes or receptors, leading to its observed biological effects. The exact pathways and targets involved depend on the specific application and the biological system being studied .
Comparison with Similar Compounds
3,4-Dichloro-5-(cyclohexylmethoxy)pyridazine can be compared with other similar compounds, such as:
3,4-Dichloropyridazine: This compound is a precursor in the synthesis of this compound and shares some chemical properties.
5-(Cyclohexylmethoxy)pyridazine: This compound lacks the chlorine atoms present in this compound, leading to different chemical reactivity and biological activities.
Other Pyridazine Derivatives: Various pyridazine derivatives with different substituents exhibit a range of chemical and biological properties.
Properties
CAS No. |
1346698-18-7 |
---|---|
Molecular Formula |
C11H14Cl2N2O |
Molecular Weight |
261.14 g/mol |
IUPAC Name |
3,4-dichloro-5-(cyclohexylmethoxy)pyridazine |
InChI |
InChI=1S/C11H14Cl2N2O/c12-10-9(6-14-15-11(10)13)16-7-8-4-2-1-3-5-8/h6,8H,1-5,7H2 |
InChI Key |
VMDSPSCQVFYVFL-UHFFFAOYSA-N |
Canonical SMILES |
C1CCC(CC1)COC2=CN=NC(=C2Cl)Cl |
Origin of Product |
United States |
Disclaimer and Information on In-Vitro Research Products
Please be aware that all articles and product information presented on BenchChem are intended solely for informational purposes. The products available for purchase on BenchChem are specifically designed for in-vitro studies, which are conducted outside of living organisms. In-vitro studies, derived from the Latin term "in glass," involve experiments performed in controlled laboratory settings using cells or tissues. It is important to note that these products are not categorized as medicines or drugs, and they have not received approval from the FDA for the prevention, treatment, or cure of any medical condition, ailment, or disease. We must emphasize that any form of bodily introduction of these products into humans or animals is strictly prohibited by law. It is essential to adhere to these guidelines to ensure compliance with legal and ethical standards in research and experimentation.