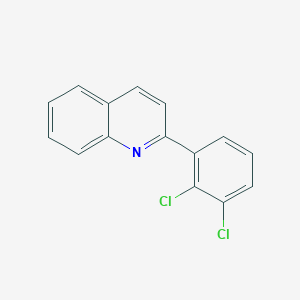
2-(2,3-Dichlorophenyl)quinoline
- Click on QUICK INQUIRY to receive a quote from our team of experts.
- With the quality product at a COMPETITIVE price, you can focus more on your research.
Overview
Description
2-(2,3-Dichlorophenyl)quinoline is a chemical compound that belongs to the quinoline family. Quinolines are aromatic compounds consisting of a benzene ring fused with a pyridine ring. This specific compound features a dichlorophenyl group attached to the quinoline structure, making it a unique derivative with distinct properties and applications.
Preparation Methods
Synthetic Routes and Reaction Conditions: The synthesis of 2-(2,3-Dichlorophenyl)quinoline typically involves the reaction of 2,3-dichlorobenzaldehyde with aniline derivatives under acidic conditions. One common method is the Pfitzinger reaction, where the aldehyde reacts with aniline in the presence of a strong acid like hydrochloric acid, followed by cyclization to form the quinoline ring.
Industrial Production Methods: Industrial production of this compound often employs similar synthetic routes but on a larger scale. The use of continuous flow reactors and optimized reaction conditions, such as temperature and pressure control, ensures high yield and purity. Catalysts like palladium on carbon may also be used to enhance the reaction efficiency.
Chemical Reactions Analysis
Types of Reactions: 2-(2,3-Dichlorophenyl)quinoline undergoes various chemical reactions, including:
Oxidation: It can be oxidized to form quinoline N-oxide derivatives.
Reduction: Reduction reactions can convert it into dihydroquinoline derivatives.
Substitution: Halogenation, nitration, and sulfonation are common substitution reactions it undergoes.
Common Reagents and Conditions:
Oxidation: Reagents like hydrogen peroxide or m-chloroperbenzoic acid.
Reduction: Catalysts such as palladium on carbon or sodium borohydride.
Substitution: Halogenating agents like chlorine or bromine, nitrating agents like nitric acid, and sulfonating agents like sulfuric acid.
Major Products:
Oxidation: Quinoline N-oxide derivatives.
Reduction: Dihydroquinoline derivatives.
Substitution: Halogenated, nitrated, or sulfonated quinoline derivatives.
Scientific Research Applications
2-(2,3-Dichlorophenyl)quinoline has a wide range of applications in scientific research:
Chemistry: Used as a building block for synthesizing more complex quinoline derivatives.
Biology: Studied for its potential biological activities, including antimicrobial and anticancer properties.
Medicine: Investigated for its potential as a therapeutic agent in treating various diseases.
Industry: Utilized in the production of dyes, pigments, and other industrial chemicals.
Mechanism of Action
The mechanism of action of 2-(2,3-Dichlorophenyl)quinoline involves its interaction with specific molecular targets and pathways. It can inhibit enzymes like DNA gyrase and topoisomerase, leading to the disruption of DNA replication and cell division. This mechanism is particularly relevant in its antimicrobial and anticancer activities.
Comparison with Similar Compounds
2-Phenylquinoline: Lacks the dichloro substitution, resulting in different chemical and biological properties.
2-(2,4-Dichlorophenyl)quinoline: Similar structure but with different chlorine substitution, leading to variations in reactivity and applications.
2-(2,3-Dichlorophenyl)pyridine: A pyridine derivative with similar substitution but different ring structure.
Uniqueness: 2-(2,3-Dichlorophenyl)quinoline stands out due to its specific dichloro substitution pattern, which imparts unique chemical reactivity and biological activity. This makes it a valuable compound for various research and industrial applications.
Properties
Molecular Formula |
C15H9Cl2N |
---|---|
Molecular Weight |
274.1 g/mol |
IUPAC Name |
2-(2,3-dichlorophenyl)quinoline |
InChI |
InChI=1S/C15H9Cl2N/c16-12-6-3-5-11(15(12)17)14-9-8-10-4-1-2-7-13(10)18-14/h1-9H |
InChI Key |
FMNHKVIFONHWFE-UHFFFAOYSA-N |
Canonical SMILES |
C1=CC=C2C(=C1)C=CC(=N2)C3=C(C(=CC=C3)Cl)Cl |
Origin of Product |
United States |
Disclaimer and Information on In-Vitro Research Products
Please be aware that all articles and product information presented on BenchChem are intended solely for informational purposes. The products available for purchase on BenchChem are specifically designed for in-vitro studies, which are conducted outside of living organisms. In-vitro studies, derived from the Latin term "in glass," involve experiments performed in controlled laboratory settings using cells or tissues. It is important to note that these products are not categorized as medicines or drugs, and they have not received approval from the FDA for the prevention, treatment, or cure of any medical condition, ailment, or disease. We must emphasize that any form of bodily introduction of these products into humans or animals is strictly prohibited by law. It is essential to adhere to these guidelines to ensure compliance with legal and ethical standards in research and experimentation.