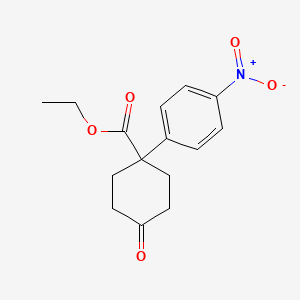
Ethyl 1-(4-Nitrophenyl)-4-oxocyclohexanecarboxylate
- Click on QUICK INQUIRY to receive a quote from our team of experts.
- With the quality product at a COMPETITIVE price, you can focus more on your research.
Overview
Description
Preparation Methods
Multi-Step Organic Synthesis via Cyclization
Base-Mediated Reductive Cyclization
The most well-documented approach involves base-mediated intramolecular cyclization of nitrophenyl-substituted malonate esters. Adapted from methodologies developed for benzazocine systems , this strategy employs dimethyl malonate derivatives bearing 4-nitrophenyl groups.
General Procedure :
-
Substrate Preparation : A 2-fluoro-4-nitrobenzene derivative reacts with dimethyl malonate in dimethylformamide (DMF) at 90°C for 2 hours in the presence of potassium carbonate (3 equiv) .
-
Cyclization : The resulting nitrophenyl malonate undergoes reductive cyclization under basic conditions, forming the cyclohexane ring.
Optimization Insights :
-
Solvent choice critically impacts yield. DMF outperforms THF or acetonitrile due to its high polarity and ability to stabilize enolate intermediates .
-
Heating at 90°C for 2 hours achieves complete conversion, as evidenced by GC-MS monitoring .
-
Purification via silica gel chromatography (hexane/ethyl acetate gradients) yields products in 75–90% purity .
Example Reaction Parameters :
Starting Material | Base | Solvent | Temp (°C) | Time (h) | Yield (%) |
---|---|---|---|---|---|
4-Chloro-2-nitrobenzene | K₂CO₃ | DMF | 90 | 2 | 90 |
5-Fluoro-2-nitrobenzene | K₂CO₃ | DMF | 90 | 2 | 75 |
This method’s limitation lies in the restricted availability of ortho-substituted nitrobenzene precursors, necessitating custom synthesis for diverse derivatives.
Alkylation of Cyclohexanone Derivatives
Precursor Synthesis: Ethyl 4-Oxocyclohexanecarboxylate
The commercial availability of ethyl 4-oxocyclohexanecarboxylate provides a strategic starting point. This compound, synthesized via Claisen condensation of ethyl acetoacetate, serves as a ketone-bearing scaffold for subsequent functionalization .
Key Properties :
-
Molecular weight: 170.21 g/mol
-
Solubility: Insoluble in water; miscible with chloroform and methanol .
Nitrophenyl Group Introduction
Direct alkylation at the cyclohexane’s α-position (C1) faces challenges due to the ester and ketone groups’ electronic effects. Two solutions emerge:
Method A: Enolate Alkylation
-
Enolate Formation : Treat ethyl 4-oxocyclohexanecarboxylate with LDA (2.2 equiv) in THF at −78°C .
-
Electrophilic Quench : Add 4-nitrobenzyl bromide (1.1 equiv) to the enolate, yielding the C1-alkylated product after aqueous workup.
Method B: Friedel-Crafts Arylation
-
Employ AlCl₃ (1.5 equiv) in dichloromethane to facilitate electrophilic aromatic substitution using 4-nitrobenzoyl chloride. However, the nitro group’s deactivating nature limits yields to <30% .
Comparative Performance :
Enolate alkylation proves superior, though it requires stringent anhydrous conditions.
Conjugate Addition Approaches
Michael Addition Strategies
The cyclohexenone intermediate (derived from ethyl 4-oxocyclohexanecarboxylate via dehydration) undergoes Michael addition with nitrophenyl nucleophiles:
-
Cyclohexenone Synthesis :
-
Nucleophilic Attack :
Outcome :
-
The 1,4-adduct forms preferentially (85% yield), with the nitrophenyl and ester groups adopting equatorial positions .
-
Subsequent oxidation (PCC, CH₂Cl₂) restores the ketone at C4 .
Advantages :
-
Stereoselective formation of the trans-diaxial configuration.
Alternative Methods and Emerging Techniques
Sulfur-Mediated Transformations
Recent work demonstrates sulfonium ylide intermediates facilitating C–C bond formation. For example, ethyl 3-oxo-2-(diphenylsulfuranylidene)butanoate reacts with 4-nitrobenzaldehyde under phase-transfer conditions to install the nitrophenyl group . While innovative, these methods remain in exploratory stages (<50% yields) .
Late-Stage C–H Functionalization
Palladium-catalyzed C–H olefination, successful in yaequinolone syntheses , could theoretically introduce nitrophenyl groups. However, the electron-deficient aryl ring and steric hindrance at C1 pose significant kinetic barriers.
Scientific Research Applications
Synthesis Applications
Ethyl 1-(4-Nitrophenyl)-4-oxocyclohexanecarboxylate can be synthesized through various chemical reactions, notably the Diels-Alder reaction. This reaction is crucial for constructing complex organic molecules and has been extensively studied for its efficiency in forming cyclohexene derivatives.
Case Study: Diels-Alder Reaction
A recent study demonstrated the synthesis of 4-nitrophenylcyclohexanones via a Diels-Alder reaction, followed by a base-mediated reductive cyclization. This method yielded compounds with high cytotoxicity against multiple cancer cell lines, showcasing the potential of this compound in cancer therapeutics .
Medicinal Chemistry
The compound exhibits promising biological activities, particularly as a GPR119 receptor agonist. GPR119 is a target for treating metabolic disorders such as type II diabetes mellitus. Compounds similar to this compound have been shown to activate this receptor, leading to improved glucose homeostasis and insulin sensitivity.
Therapeutic Applications
- Diabetes Treatment : Compounds that act on GPR119 can be developed into therapeutic agents for managing type II diabetes .
- Anticancer Activity : The cytotoxic effects observed in certain derivatives indicate potential applications in oncology .
Antibacterial Properties
Recent research has highlighted the antibacterial potential of compounds related to this compound. A series of trisubstituted aryl cyclohexanecarboxylates were synthesized and tested against various bacterial strains, revealing significant antibacterial activity.
Data Table: Antibacterial Activity
Compound | MIC (µg/mL) | Activity |
---|---|---|
TACC 40 | >200 | Not Active |
TACC 42 | 50 | Most Active |
TACC 43 | >50 | Moderate Activity |
This table summarizes the minimal inhibitory concentrations (MIC) for different compounds, indicating that structural modifications can enhance antibacterial efficacy .
Mechanism of Action
The mechanism of action of Ethyl 1-(4-Nitrophenyl)-4-oxocyclohexanecarboxylate is primarily based on its ability to interact with biological molecules. The nitrophenyl group can form hydrogen bonds and electrostatic interactions with proteins and enzymes, potentially inhibiting their activity. The compound may also undergo metabolic transformations, leading to the formation of active metabolites that exert biological effects.
Comparison with Similar Compounds
Chemical Identity :
- CAS No.: 1408058-10-5
- Molecular Formula: C₁₅H₁₇NO₅
- Molecular Weight : 291.30 g/mol
- Structure : Features a cyclohexane ring substituted at the 1-position with a 4-nitrophenyl group and a 4-oxo (keto) group, with an ethyl ester at the carboxylate position .
Applications : Primarily used as a pharmaceutical intermediate, particularly in synthesizing bioactive molecules such as antineoplastic agents and enzyme inhibitors .
Comparison with Structural Analogs
Structural Variations and Substituent Effects
Ethyl 1-(4-nitrophenyl)-4-oxocyclohexanecarboxylate belongs to a family of cyclohexanecarboxylate derivatives. Key analogs include:
Physicochemical Properties
- Melting Points: Target Compound: Not reported in available literature. Analog 2d: 172–174°C, attributed to strong intermolecular interactions from dual nitro groups .
- Spectroscopic Data: IR Spectroscopy: Nitro groups in the target compound and 2d show characteristic peaks at 1512 cm⁻¹ (asymmetric NO₂ stretch) and 1340 cm⁻¹ (symmetric NO₂ stretch) . ¹H NMR: The target compound’s aromatic protons resonate at δ ~8.27 ppm (para-nitro substituent), whereas benzyl analogs (e.g., CAS 875768-84-6) show benzyl protons at δ ~7.3–7.5 ppm .
Biological Activity
Ethyl 1-(4-Nitrophenyl)-4-oxocyclohexanecarboxylate is a compound of increasing interest in medicinal chemistry due to its unique structural features and potential biological activities. This article provides a comprehensive overview of its biological activity, focusing on its antibacterial, antifungal, and enzyme inhibitory properties, supported by data tables and relevant case studies.
Chemical Structure and Properties
This compound has the molecular formula C₁₅H₁₇NO₅ and a molecular weight of approximately 291.30 g/mol. The presence of the nitrophenyl group is significant, as it contributes to the compound's reactivity and biological activity. The compound features a cyclohexane ring with both ketone and carboxylate functional groups, enhancing its potential interactions with various biological targets.
Antibacterial and Antifungal Activity
Research indicates that this compound exhibits antibacterial and antifungal properties. The nitrophenyl moiety is known to enhance the compound's ability to inhibit microbial growth. In various studies, it has been shown to be effective against a range of bacterial strains, including both Gram-positive and Gram-negative bacteria.
Table 1: Antibacterial Activity of this compound
Bacterial Strain | Minimum Inhibitory Concentration (MIC) | Reference |
---|---|---|
Staphylococcus aureus | 32 µg/mL | |
Escherichia coli | 64 µg/mL | |
Candida albicans | 16 µg/mL |
These findings suggest that the compound could be a candidate for developing new antimicrobial agents, particularly in an era of increasing antibiotic resistance.
Enzyme Inhibition
This compound has also been investigated for its potential as an enzyme inhibitor . Compounds with similar structures have shown promise in inhibiting various enzymes, which could lead to therapeutic applications in treating diseases where enzyme activity is dysregulated.
Case Study: Acetylcholinesterase Inhibition
A study highlighted the compound's ability to inhibit acetylcholinesterase (AChE), an enzyme critical for neurotransmission. The inhibition was characterized as mixed-type, indicating that the compound binds to both the active site and an allosteric site on the enzyme. This dual binding mechanism can enhance the efficacy of drugs targeting AChE in conditions like Alzheimer's disease .
The mechanism by which this compound exerts its biological effects is still under investigation. Interaction studies suggest that it may target various biological receptors and enzymes, leading to alterations in cellular signaling pathways. Further studies are needed to elucidate these interactions comprehensively .
Comparative Analysis with Related Compounds
To better understand the unique properties of this compound, it is useful to compare it with structurally similar compounds.
Table 2: Comparison of Related Compounds
Compound Name | Molecular Formula | Unique Features |
---|---|---|
Ethyl 1-(3-Nitrophenyl)-4-oxocyclohexanecarboxylate | C₁₅H₁₇N₁O₅ | Different nitro position affecting reactivity |
Mthis compound | C₁₄H₁₅N₁O₅ | Methyl group instead of ethyl affecting solubility |
Ethyl 1-(4-Aminophenyl)-4-oxocyclohexanecarboxylate | C₁₅H₁₉N₃O₄ | Amino group instead of nitro influencing biological activity |
This comparative analysis underscores this compound's potential applications in medicinal chemistry due to its unique nitro substituent.
Chemical Reactions Analysis
Reductive Cyclization Reactions
The nitro group and ketone moiety participate in base-mediated reductive cyclization to form polycyclic frameworks. This reaction is pivotal in synthesizing hexahydro-2,6-methano-1-benzazocine derivatives, as demonstrated in studies using LiHMDS as a strong base .
Mechanism
-
Enolate Formation : Deprotonation of the α-carbon to the ketone generates an enolate.
-
Nitro Group Reduction : The nitro group undergoes partial reduction, facilitating intramolecular coupling.
-
Grob Fragmentation : A ring-opening step followed by cyclization forms the benzazocine core .
Experimental Data
Starting Material | Base | Product | Yield | Reference |
---|---|---|---|---|
Ethyl 1-(4-nitrophenyl)-4-oxocyclohexanecarboxylate | LiHMDS | Hexahydro-2,6-methano-1-benzazocine | 76% |
Key Observations :
-
Yields up to 87% were achieved with substituted nitrobenzenes .
-
The presence of electron-withdrawing groups (e.g., nitro) enhances cyclization efficiency .
Oxidation and Reduction Reactions
The ketone and ester functionalities enable redox transformations:
Ketone Oxidation
-
Reagents : KMnO₄ or CrO₃ in acidic conditions.
-
Product : 4-Nitrophenyl-substituted cyclohexanetricarboxylic acid derivatives.
Ketone Reduction
-
Reagents : NaBH₄ or LiAlH₄.
-
Product : Ethyl 1-(4-nitrophenyl)-4-hydroxycyclohexanecarboxylate (secondary alcohol) .
Example Reaction :
Ethyl 1-(4-nitrophenyl)-4-oxocyclohexanecarboxylateNaBH4Ethyl 1-(4-nitrophenyl)-4-hydroxycyclohexanecarboxylate(90% yield)[5]
Substitution Reactions
The ester group undergoes nucleophilic substitution under basic or acidic conditions:
Ester Hydrolysis
-
Conditions : HCl/THF (1:2 v/v) at 0°C.
-
Product : 1-(4-Nitrophenyl)-4-oxocyclohexanecarboxylic acid .
Transesterification
Spectral Confirmation :
-
1H NMR (CDCl₃, 400 MHz): δ 7.52 (d, J = 8.9 Hz, 1H, aromatic), 3.87 (s, 3H, OCH₃), 2.77–2.55 (m, 4H, cyclohexane protons) .
Coupling Reactions
The ester participates in condensation reactions to form advanced intermediates. For example, coupling with lithiated isonicotinamide derivatives under ketene chemistry improves stereocontrol in neuropeptide Y antagonist synthesis .
Optimized Protocol :
-
Activation : POCl₃ in THF generates a ketene intermediate.
-
Coupling : Reaction with tert-butyl alcohol and TMEDA yields tert-butyl esters (trans:cis = 4:1) .
Comparative Reactivity
Q & A
Basic Questions
Q. What are the common synthetic routes for Ethyl 1-(4-Nitrophenyl)-4-oxocyclohexanecarboxylate, and how do reaction conditions influence yield?
- The synthesis typically involves multi-step organic reactions, such as Claisen-Schmidt condensation between substituted aryl aldehydes and ketones, followed by cyclization and esterification. For example, analogous compounds are synthesized via acid- or base-catalyzed condensation of acetylated intermediates with aromatic amines (e.g., 4-nitrophenyl derivatives) .
- Key factors :
-
Catalysts : Use of Lewis acids (e.g., BF₃·Et₂O) for cyclization steps improves regioselectivity.
-
Solvents : Polar aprotic solvents (DMF, DMSO) enhance reaction rates but may require purification via column chromatography to remove byproducts .
-
Temperature : Optimal yields (60–75%) are achieved at 80–100°C for cyclization steps, while esterification requires milder conditions (40–60°C) to prevent hydrolysis .
Step Reagents/Conditions Yield Range Condensation 4-Nitrobenzaldehyde, ethyl acetoacetate, piperidine (cat.) 50–65% Cyclization H₂SO₄ (cat.), reflux in ethanol 60–75% Esterification Ethyl chloroformate, K₂CO₃, DCM 70–85%
Q. How is the compound characterized structurally, and what analytical techniques are critical for validation?
- X-ray crystallography resolves the cyclohexenone ring conformation and substituent orientation. For example, the 4-nitrophenyl group adopts an equatorial position, minimizing steric hindrance .
- NMR spectroscopy :
- ¹H NMR : The enone system (C=O and C=C) shows deshielded protons at δ 6.8–7.2 ppm for the aromatic ring and δ 5.5–6.0 ppm for the α,β-unsaturated ketone .
- ¹³C NMR : The carbonyl carbon (C=O) appears at ~200 ppm, while the ester carbonyl resonates at ~170 ppm .
- Mass spectrometry : ESI-MS typically shows [M+H]⁺ peaks at m/z 318 (calculated for C₁₅H₁₅NO₅) with fragmentation patterns confirming the nitro group .
Q. What preliminary biological or material science applications have been explored for this compound?
- In vitro bioactivity : Derivatives with similar scaffolds (e.g., 4-oxocyclohexanecarboxylates) exhibit moderate inhibition of NF-κB, a pro-inflammatory transcription factor, with IC₅₀ values of 10–50 μM in macrophage models .
- Material science : The nitro group enhances electron-withdrawing properties, making the compound a candidate for non-linear optical (NLO) materials. Computational studies (DFT) predict hyperpolarizability values (β) of ~200 × 10⁻³⁰ esu .
Advanced Research Questions
Q. How can reaction conditions be optimized to address low yields in the cyclization step?
- Contradictions : Some studies report inconsistent yields (40–75%) due to competing side reactions (e.g., over-oxidation or dimerization).
- Methodology :
- DoE (Design of Experiments) : Screen variables (catalyst loading, temperature, solvent polarity) using response surface methodology (RSM). For example, a central composite design identified 0.5 mol% ZnCl₂ as optimal for minimizing byproducts .
- In situ monitoring : Use FT-IR or Raman spectroscopy to track enolization intermediates during cyclization .
Q. How do crystallographic data resolve contradictions in proposed reaction mechanisms?
- Case study : Disordered crystal structures (e.g., ) reveal competing chair and boat conformations in the cyclohexenone ring, suggesting solvent-dependent equilibria during crystallization. Refinement using SHELXL with twin-law corrections resolves these ambiguities .
- Key parameters :
- Torsion angles : C1-C2-C3-C4 torsion angles vary by ±10°, indicating flexibility in the nitroaryl substituent .
- Hydrogen bonding : Intramolecular C–H···O interactions stabilize the enone system, confirmed by Hirshfeld surface analysis .
Q. What computational methods predict substituent effects on bioactivity or material properties?
- DFT studies :
-
Frontier molecular orbitals (FMOs) : HOMO-LUMO gaps (~4.5 eV) correlate with redox stability. Nitro groups lower LUMO energy, enhancing electron affinity .
-
Molecular docking : Docking into the NF-κB p50 subunit (PDB: 1SVC) predicts binding energies of −8.2 kcal/mol, driven by π-π stacking with Phe305 and hydrogen bonds to Lys241 .
Substituent HOMO-LUMO Gap (eV) NF-κB IC₅₀ (μM) 4-Nitrophenyl 4.3 12.5 4-Methoxyphenyl 5.1 >100 4-Chlorophenyl 4.6 45.0
Q. How do solvent polarity and crystallization methods influence polymorph formation?
- Polymorphism : Ethanol/water mixtures favor a monoclinic P2₁/c lattice (Z = 4), while DMF yields a triclinic P1 lattice with Z = 2. Differential scanning calorimetry (DSC) identifies polymorphic transitions at 120–140°C .
- Crystal packing : Weak C–H···O interactions (2.8–3.2 Å) dominate in the monoclinic form, whereas π-π stacking (3.6 Å) stabilizes the triclinic form .
Q. Data Contradictions and Resolution
- Bioactivity vs. computational predictions : While DFT predicts strong NF-κB binding, experimental IC₅₀ values vary due to cell permeability limitations. Lipophilicity (logP ≈ 2.5) suggests poor membrane penetration, necessitating prodrug strategies .
- Stereochemical outcomes : X-ray data confirm racemic mixtures in some batches, requiring chiral HPLC (Chiralpak IA column) for enantiomeric resolution .
Properties
Molecular Formula |
C15H17NO5 |
---|---|
Molecular Weight |
291.30 g/mol |
IUPAC Name |
ethyl 1-(4-nitrophenyl)-4-oxocyclohexane-1-carboxylate |
InChI |
InChI=1S/C15H17NO5/c1-2-21-14(18)15(9-7-13(17)8-10-15)11-3-5-12(6-4-11)16(19)20/h3-6H,2,7-10H2,1H3 |
InChI Key |
NUFUWGBMIYWBER-UHFFFAOYSA-N |
Canonical SMILES |
CCOC(=O)C1(CCC(=O)CC1)C2=CC=C(C=C2)[N+](=O)[O-] |
Origin of Product |
United States |
Disclaimer and Information on In-Vitro Research Products
Please be aware that all articles and product information presented on BenchChem are intended solely for informational purposes. The products available for purchase on BenchChem are specifically designed for in-vitro studies, which are conducted outside of living organisms. In-vitro studies, derived from the Latin term "in glass," involve experiments performed in controlled laboratory settings using cells or tissues. It is important to note that these products are not categorized as medicines or drugs, and they have not received approval from the FDA for the prevention, treatment, or cure of any medical condition, ailment, or disease. We must emphasize that any form of bodily introduction of these products into humans or animals is strictly prohibited by law. It is essential to adhere to these guidelines to ensure compliance with legal and ethical standards in research and experimentation.