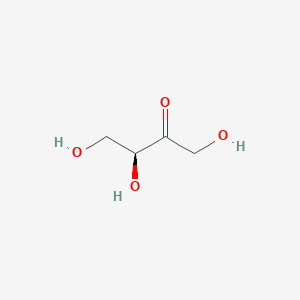
L-Erythrulose
Overview
Description
L-Erythrulose is a tetrose carbohydrate with the chemical formula C₄H₈O₄. It is a ketose, meaning it contains a ketone group. This compound naturally occurs in red raspberries and is commonly used in self-tanning products due to its ability to react with amino acids in the skin, producing a browning effect similar to that of dihydroxyacetone .
Mechanism of Action
- L-Erythrulose, also known as erythrulose, is a tetrose carbohydrate with the chemical formula C₄H₈O₄ .
- The tan produced by erythrulose/DHA has an SPF of up to 3 and enhances free radical injury from UV exposure for 24 hours after application .
Target of Action
Mode of Action
Biochemical Pathways
Action Environment
Biochemical Analysis
Biochemical Properties
L-Erythrulose can be metabolized in some reversible metabolic reactions such as dehydrogenation to D- or this compound by NAD-dependent cytoplasmic polyol dehydrogenase . It can also be phosphorylated by glycerol kinase to erythritol-1-phosphate followed by dehydrogenation to D-erythrulose 1-phosphate via α–glycerophosphate dehydrogenase .
Cellular Effects
It is known that this compound can be produced by the oxidation of meso-erythritol . This oxidation process is catalyzed by membrane-bound dehydrogenases in Gluconobacter oxydans , suggesting that this compound might play a role in the oxidative stress response in cells.
Molecular Mechanism
The molecular mechanism of this compound involves its interaction with enzymes such as NAD-dependent cytoplasmic polyol dehydrogenase and glycerol kinase . These enzymes catalyze the conversion of this compound to other metabolites, influencing cellular metabolism .
Temporal Effects in Laboratory Settings
It is known that this compound can be produced efficiently by Gluconobacter oxydans in a DO-controlled stirred-tank bioreactor , suggesting that this compound is stable under these conditions.
Metabolic Pathways
This compound is involved in the pentose phosphate pathway . It can be converted to D- or this compound by NAD-dependent cytoplasmic polyol dehydrogenase . It can also be phosphorylated by glycerol kinase to erythritol-1-phosphate .
Subcellular Localization
Given that this compound is involved in metabolic pathways that occur in the cytoplasm , it is likely that this compound is localized in the cytoplasm of cells.
Preparation Methods
Synthetic Routes and Reaction Conditions: L-Erythrulose can be synthesized through the oxidation of meso-erythritol. This process is often catalyzed by membrane-bound dehydrogenases in bacteria such as Gluconobacter oxydans. The oxidation reaction is typically carried out in a controlled environment, with specific conditions such as temperature and pH being carefully monitored to optimize yield .
Industrial Production Methods: In industrial settings, this compound is produced using a continuous membrane bioreactor with full cell retention. This method helps to overcome substrate inhibition and achieve high product yields. For example, a study reported achieving a product concentration of 54 g/L this compound with a space-time yield of 27 g/L/h .
Chemical Reactions Analysis
Types of Reactions: L-Erythrulose undergoes various chemical reactions, including:
Oxidation: Conversion of meso-erythritol to this compound.
Reduction: this compound can be reduced back to meso-erythritol under specific conditions.
Substitution: It can participate in substitution reactions, forming various derivatives.
Common Reagents and Conditions:
Oxidation: Catalyzed by pyrroloquinoline quinone-containing dehydrogenases in the presence of oxygen.
Reduction: Requires NADH as a coenzyme and is typically carried out at a pH of 6.0.
Major Products:
Oxidation: this compound.
Reduction: Meso-erythritol.
Scientific Research Applications
L-Erythrulose has a wide range of applications in scientific research:
Chemistry: Used as a starting material for the synthesis of various chemical compounds.
Biology: Studied for its role in metabolic pathways and its interactions with enzymes.
Medicine: Investigated for its potential therapeutic effects and its use in drug delivery systems.
Industry: Widely used in the cosmetics industry, particularly in self-tanning products.
Comparison with Similar Compounds
- Dihydroxyacetone (DHA)
- Erythritol
- Xylitol
Properties
IUPAC Name |
(3S)-1,3,4-trihydroxybutan-2-one | |
---|---|---|
Source | PubChem | |
URL | https://pubchem.ncbi.nlm.nih.gov | |
Description | Data deposited in or computed by PubChem | |
InChI |
InChI=1S/C4H8O4/c5-1-3(7)4(8)2-6/h3,5-7H,1-2H2/t3-/m0/s1 | |
Source | PubChem | |
URL | https://pubchem.ncbi.nlm.nih.gov | |
Description | Data deposited in or computed by PubChem | |
InChI Key |
UQPHVQVXLPRNCX-VKHMYHEASA-N | |
Source | PubChem | |
URL | https://pubchem.ncbi.nlm.nih.gov | |
Description | Data deposited in or computed by PubChem | |
Canonical SMILES |
C(C(C(=O)CO)O)O | |
Source | PubChem | |
URL | https://pubchem.ncbi.nlm.nih.gov | |
Description | Data deposited in or computed by PubChem | |
Isomeric SMILES |
C([C@@H](C(=O)CO)O)O | |
Source | PubChem | |
URL | https://pubchem.ncbi.nlm.nih.gov | |
Description | Data deposited in or computed by PubChem | |
Molecular Formula |
C4H8O4 | |
Source | PubChem | |
URL | https://pubchem.ncbi.nlm.nih.gov | |
Description | Data deposited in or computed by PubChem | |
DSSTOX Substance ID |
DTXSID801019042 | |
Record name | (3S)-1,3,4-Trihydroxy-2-butanone | |
Source | EPA DSSTox | |
URL | https://comptox.epa.gov/dashboard/DTXSID801019042 | |
Description | DSSTox provides a high quality public chemistry resource for supporting improved predictive toxicology. | |
Molecular Weight |
120.10 g/mol | |
Source | PubChem | |
URL | https://pubchem.ncbi.nlm.nih.gov | |
Description | Data deposited in or computed by PubChem | |
Physical Description |
Solid | |
Record name | L-Erythrulose | |
Source | Human Metabolome Database (HMDB) | |
URL | http://www.hmdb.ca/metabolites/HMDB0006293 | |
Description | The Human Metabolome Database (HMDB) is a freely available electronic database containing detailed information about small molecule metabolites found in the human body. | |
Explanation | HMDB is offered to the public as a freely available resource. Use and re-distribution of the data, in whole or in part, for commercial purposes requires explicit permission of the authors and explicit acknowledgment of the source material (HMDB) and the original publication (see the HMDB citing page). We ask that users who download significant portions of the database cite the HMDB paper in any resulting publications. | |
CAS No. |
533-50-6 | |
Record name | L-Erythrulose | |
Source | CAS Common Chemistry | |
URL | https://commonchemistry.cas.org/detail?cas_rn=533-50-6 | |
Description | CAS Common Chemistry is an open community resource for accessing chemical information. Nearly 500,000 chemical substances from CAS REGISTRY cover areas of community interest, including common and frequently regulated chemicals, and those relevant to high school and undergraduate chemistry classes. This chemical information, curated by our expert scientists, is provided in alignment with our mission as a division of the American Chemical Society. | |
Explanation | The data from CAS Common Chemistry is provided under a CC-BY-NC 4.0 license, unless otherwise stated. | |
Record name | L-Erythrulose | |
Source | ChemIDplus | |
URL | https://pubchem.ncbi.nlm.nih.gov/substance/?source=chemidplus&sourceid=0000533506 | |
Description | ChemIDplus is a free, web search system that provides access to the structure and nomenclature authority files used for the identification of chemical substances cited in National Library of Medicine (NLM) databases, including the TOXNET system. | |
Record name | (3S)-1,3,4-Trihydroxy-2-butanone | |
Source | EPA DSSTox | |
URL | https://comptox.epa.gov/dashboard/DTXSID801019042 | |
Description | DSSTox provides a high quality public chemistry resource for supporting improved predictive toxicology. | |
Record name | L-ERYTHRULOSE | |
Source | FDA Global Substance Registration System (GSRS) | |
URL | https://gsrs.ncats.nih.gov/ginas/app/beta/substances/WKK1W5B83O | |
Description | The FDA Global Substance Registration System (GSRS) enables the efficient and accurate exchange of information on what substances are in regulated products. Instead of relying on names, which vary across regulatory domains, countries, and regions, the GSRS knowledge base makes it possible for substances to be defined by standardized, scientific descriptions. | |
Explanation | Unless otherwise noted, the contents of the FDA website (www.fda.gov), both text and graphics, are not copyrighted. They are in the public domain and may be republished, reprinted and otherwise used freely by anyone without the need to obtain permission from FDA. Credit to the U.S. Food and Drug Administration as the source is appreciated but not required. | |
Record name | L-Erythrulose | |
Source | Human Metabolome Database (HMDB) | |
URL | http://www.hmdb.ca/metabolites/HMDB0006293 | |
Description | The Human Metabolome Database (HMDB) is a freely available electronic database containing detailed information about small molecule metabolites found in the human body. | |
Explanation | HMDB is offered to the public as a freely available resource. Use and re-distribution of the data, in whole or in part, for commercial purposes requires explicit permission of the authors and explicit acknowledgment of the source material (HMDB) and the original publication (see the HMDB citing page). We ask that users who download significant portions of the database cite the HMDB paper in any resulting publications. | |
Retrosynthesis Analysis
AI-Powered Synthesis Planning: Our tool employs the Template_relevance Pistachio, Template_relevance Bkms_metabolic, Template_relevance Pistachio_ringbreaker, Template_relevance Reaxys, Template_relevance Reaxys_biocatalysis model, leveraging a vast database of chemical reactions to predict feasible synthetic routes.
One-Step Synthesis Focus: Specifically designed for one-step synthesis, it provides concise and direct routes for your target compounds, streamlining the synthesis process.
Accurate Predictions: Utilizing the extensive PISTACHIO, BKMS_METABOLIC, PISTACHIO_RINGBREAKER, REAXYS, REAXYS_BIOCATALYSIS database, our tool offers high-accuracy predictions, reflecting the latest in chemical research and data.
Strategy Settings
Precursor scoring | Relevance Heuristic |
---|---|
Min. plausibility | 0.01 |
Model | Template_relevance |
Template Set | Pistachio/Bkms_metabolic/Pistachio_ringbreaker/Reaxys/Reaxys_biocatalysis |
Top-N result to add to graph | 6 |
Feasible Synthetic Routes
Q1: What is the molecular formula and weight of L-erythrulose?
A1: this compound has a molecular formula of C4H8O4 and a molecular weight of 120.10 g/mol.
Q2: Are there any specific spectroscopic data available for this compound?
A2: Yes, studies have utilized techniques like 13C-NMR [] and polarimetry [] to characterize this compound, confirming its structure and properties.
Q3: What is known about the stability of this compound?
A3: Research indicates that the stability of this compound can be impacted by factors like pH and temperature. For instance, one study observed that an erythrose reductase, which acts on this compound, exhibited stability within a pH range of 6.0-8.0 and below 40°C [].
Q4: How is this compound produced through microbial activity?
A4: Several studies highlight the production of this compound through the fermentation of erythritol by various microorganisms. For example, Gluconobacter frateurii CHM 43 efficiently oxidizes erythritol to this compound []. Similarly, Gluconobacter kondonii HD385 produced a significant yield of 82.9 g/L this compound from a 10% erythritol medium [].
Q5: What role does this compound play in the metabolism of erythritol by bacteria like Propionibacterium pentosaceum?
A5: Research suggests that this compound-1-phosphate is a key intermediate in the erythritol metabolic pathway of P. pentosaceum. It is believed to be formed from L-erythritol-1-phosphate and subsequently cleaved between the C3 and C4 positions, yielding formaldehyde and dihydroxyacetone-phosphate [].
Q6: Can this compound be produced using enzymes?
A6: Yes, this compound can be synthesized enzymatically. One approach utilizes a three-enzyme cascade involving D-amino acid oxidase, catalase, and transketolase, with D-serine and glycolaldehyde as starting materials []. Another method leverages a polyol dehydrogenase from the yeast Lipomyces starkeyi CBS 1807, which specifically oxidizes erythritol to this compound [].
Q7: How does this compound interact with enzymes like ribulokinase?
A8: this compound acts as a competitive inhibitor of L-ribulose for the enzyme ribulokinase. This competition influences the enzyme's kinetic mechanism and substrate specificity [].
Q8: Can this compound be used in the synthesis of rare sugars?
A9: Yes, the enzyme L-arabinose isomerase from Mycobacterium smegmatis SMDU has demonstrated the ability to catalyze the isomerization of this compound to L-threose. This enzymatic conversion holds potential for producing the rare sugar L-threose from the more readily available erythritol [].
Q9: How do structural modifications of this compound derivatives impact their reactivity?
A10: Studies exploring the diastereoselectivity of organometallic additions to protected this compound derivatives, modified with O-silyl, O-benzyl, and O-trityl groups, suggest that the position and nature of these protecting groups significantly influence stereochemical outcomes [].
Q10: What is the significance of alpha-chelation in reactions involving this compound derivatives?
A11: Research indicates that the formation of alpha-chelates plays a crucial role in the stereochemical control of nucleophilic additions to alpha,beta-dioxygenated ketones derived from this compound []. This understanding is valuable in designing stereoselective synthetic strategies.
Q11: Have there been any computational studies on this compound and its derivatives?
A12: Yes, theoretical studies using methods like PM3 and HF/3-21G have been conducted to investigate the addition of organomagnesium reagents to chiral alpha-alkoxy carbonyl compounds, including this compound derivatives. These studies offer valuable insights into the reaction mechanisms and stereochemistry of these reactions [].
Q12: What is the historical context of this compound research?
A13: Early research recognized the ability of Acetobacter suboxydans to oxidize erythritol to this compound []. Over time, studies have explored its role in metabolic pathways [], enzymatic synthesis [], and applications in biocatalysis [].
Disclaimer and Information on In-Vitro Research Products
Please be aware that all articles and product information presented on BenchChem are intended solely for informational purposes. The products available for purchase on BenchChem are specifically designed for in-vitro studies, which are conducted outside of living organisms. In-vitro studies, derived from the Latin term "in glass," involve experiments performed in controlled laboratory settings using cells or tissues. It is important to note that these products are not categorized as medicines or drugs, and they have not received approval from the FDA for the prevention, treatment, or cure of any medical condition, ailment, or disease. We must emphasize that any form of bodily introduction of these products into humans or animals is strictly prohibited by law. It is essential to adhere to these guidelines to ensure compliance with legal and ethical standards in research and experimentation.