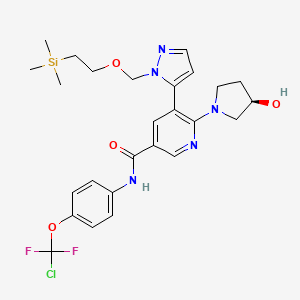
(R)-N-(4-(chlorodifluoromethoxy)phenyl)-6-(3-hydroxypyrrolidin-1-yl)-5-(1-((2-(trimethylsilyl)ethoxy)methyl)-1H-pyrazol-5-yl)nicotinamide
- Click on QUICK INQUIRY to receive a quote from our team of experts.
- With the quality product at a COMPETITIVE price, you can focus more on your research.
Overview
Description
®-N-(4-(chlorodifluoromethoxy)phenyl)-6-(3-hydroxypyrrolidin-1-yl)-5-(1-((2-(trimethylsilyl)ethoxy)methyl)-1H-pyrazol-5-yl)nicotinamide is a complex organic compound with significant potential in various scientific fields This compound features a unique combination of functional groups, including a chlorodifluoromethoxy phenyl ring, a hydroxypyrrolidinyl group, and a pyrazolyl nicotinamide moiety
Preparation Methods
Synthetic Routes and Reaction Conditions
The synthesis of ®-N-(4-(chlorodifluoromethoxy)phenyl)-6-(3-hydroxypyrrolidin-1-yl)-5-(1-((2-(trimethylsilyl)ethoxy)methyl)-1H-pyrazol-5-yl)nicotinamide typically involves multiple steps, starting from commercially available precursors. A common synthetic route includes:
Formation of the Chlorodifluoromethoxy Phenyl Intermediate: This step involves the reaction of 4-chlorophenol with chlorodifluoromethane in the presence of a base such as potassium carbonate, under reflux conditions.
Synthesis of the Pyrazolyl Nicotinamide Core: This involves the cyclization of a suitable hydrazine derivative with a nicotinic acid ester, followed by functional group modifications to introduce the trimethylsilyl ethoxy moiety.
Coupling of Intermediates: The chlorodifluoromethoxy phenyl intermediate is coupled with the pyrazolyl nicotinamide core using a palladium-catalyzed cross-coupling reaction.
Introduction of the Hydroxypyrrolidinyl Group: This final step involves the nucleophilic substitution of a suitable pyrrolidine derivative onto the coupled intermediate, under basic conditions.
Industrial Production Methods
Industrial production of this compound would likely involve optimization of the above synthetic route to maximize yield and purity while minimizing costs and environmental impact. This could include the use of continuous flow reactors for key steps, solvent recycling, and the development of more efficient catalysts.
Chemical Reactions Analysis
Types of Reactions
®-N-(4-(chlorodifluoromethoxy)phenyl)-6-(3-hydroxypyrrolidin-1-yl)-5-(1-((2-(trimethylsilyl)ethoxy)methyl)-1H-pyrazol-5-yl)nicotinamide undergoes various types of chemical reactions, including:
Oxidation: The hydroxypyrrolidinyl group can be oxidized to form corresponding ketones or carboxylic acids.
Reduction: The nitro groups, if present, can be reduced to amines.
Substitution: The chlorodifluoromethoxy group can undergo nucleophilic substitution reactions.
Common Reagents and Conditions
Oxidation: Reagents such as potassium permanganate or chromium trioxide under acidic conditions.
Reduction: Hydrogen gas with a palladium catalyst or lithium aluminum hydride.
Substitution: Nucleophiles such as amines or thiols under basic conditions.
Major Products
Oxidation: Formation of ketones or carboxylic acids.
Reduction: Formation of amines.
Substitution: Formation of substituted phenyl derivatives.
Scientific Research Applications
Chemistry
In chemistry, this compound is used as a building block for the synthesis of more complex molecules. Its unique functional groups allow for diverse chemical modifications, making it valuable in the development of new materials and catalysts.
Biology
In biological research, ®-N-(4-(chlorodifluoromethoxy)phenyl)-6-(3-hydroxypyrrolidin-1-yl)-5-(1-((2-(trimethylsilyl)ethoxy)methyl)-1H-pyrazol-5-yl)nicotinamide is studied for its potential as a biochemical probe. Its ability to interact with specific proteins and enzymes makes it useful in studying cellular processes and signaling pathways.
Medicine
In medicine, this compound is investigated for its potential therapeutic applications. Its structural features suggest it could act as an inhibitor of specific enzymes or receptors, making it a candidate for drug development in areas such as oncology and neurology.
Industry
In industry, this compound is explored for its potential use in the development of advanced materials, such as polymers and coatings, due to its unique chemical properties.
Mechanism of Action
The mechanism of action of ®-N-(4-(chlorodifluoromethoxy)phenyl)-6-(3-hydroxypyrrolidin-1-yl)-5-(1-((2-(trimethylsilyl)ethoxy)methyl)-1H-pyrazol-5-yl)nicotinamide involves its interaction with specific molecular targets, such as enzymes or receptors. The compound’s functional groups allow it to bind to active sites, inhibiting or modulating the activity of these targets. This can lead to changes in cellular processes and signaling pathways, resulting in the compound’s observed biological effects.
Comparison with Similar Compounds
Similar Compounds
®-N-(4-(chlorodifluoromethoxy)phenyl)-6-(3-hydroxypyrrolidin-1-yl)-5-(1-((2-(trimethylsilyl)ethoxy)methyl)-1H-pyrazol-5-yl)nicotinamide: Unique due to its combination of functional groups.
®-N-(4-(chlorodifluoromethoxy)phenyl)-6-(3-hydroxypyrrolidin-1-yl)-5-(1-((2-(trimethylsilyl)ethoxy)methyl)-1H-pyrazol-5-yl)nicotinamide: Similar structure but different substituents.
Uniqueness
The uniqueness of ®-N-(4-(chlorodifluoromethoxy)phenyl)-6-(3-hydroxypyrrolidin-1-yl)-5-(1-((2-(trimethylsilyl)ethoxy)methyl)-1H-pyrazol-5-yl)nicotinamide lies in its specific combination of functional groups, which confer distinct chemical reactivity and biological activity. This makes it a valuable compound for various scientific and industrial applications.
Biological Activity
The compound (R)-N-(4-(chlorodifluoromethoxy)phenyl)-6-(3-hydroxypyrrolidin-1-yl)-5-(1-((2-(trimethylsilyl)ethoxy)methyl)-1H-pyrazol-5-yl)nicotinamide, commonly known as Asciminib, is a novel drug that has garnered attention for its unique mechanism of action as a specifically targeting ABL1 myristoyl pocket (STAMP) inhibitor. This article delves into its biological activity, highlighting key research findings, case studies, and relevant data.
Property | Value |
---|---|
Molecular Formula | C20H18ClF2N5O3 |
Molecular Weight | 449.84 g/mol |
CAS Number | 1492952-76-7 |
Boiling Point | 631.7 ± 55.0 °C (Predicted) |
Density | 1.518 ± 0.06 g/cm³ (Predicted) |
Solubility | DMSO: 93.0 mg/mL; Ethanol: 90.0 mg/mL |
pKa | 10.81 ± 0.70 (Predicted) |
Asciminib operates by binding to the myristoyl site of the BCR-ABL1 protein, locking it into an inactive conformation. This mechanism is distinct from traditional tyrosine kinase inhibitors (TKIs) like imatinib, allowing it to effectively overcome drug resistance associated with ATP-binding site mutations. Specifically, it restores the natural autoinhibition of the ABL1b protein, making it particularly effective in chronic myeloid leukemia (CML) patients who have previously been treated with multiple TKIs .
Efficacy in Cancer Treatment
Asciminib has been granted accelerated approval for treating Philadelphia chromosome-positive (Ph+) CML in chronic phase, especially in patients previously treated with two or more TKIs and those with the T315I mutation. Clinical trials have demonstrated its ability to significantly reduce BCR-ABL1 levels in patients, leading to improved hematologic and cytogenetic responses .
Pharmacokinetics
The pharmacokinetic profile of Asciminib indicates:
- Elimination Half-Life : Approximately 14.2 hours
- Protein Binding : High at around 97%
These factors contribute to its sustained efficacy and manageable dosing schedule .
Case Studies
Several clinical studies have highlighted Asciminib's effectiveness:
- In a Phase I trial, patients with Ph+ CML showed a major molecular response rate of over 50% after treatment with Asciminib for 24 weeks.
- Long-term follow-up studies indicate that patients maintain responses over extended periods, showcasing the drug's potential for long-term management of CML .
Side Effects and Tolerability
While Asciminib is generally well-tolerated, some reported side effects include:
Properties
Molecular Formula |
C26H32ClF2N5O4Si |
---|---|
Molecular Weight |
580.1 g/mol |
IUPAC Name |
N-[4-[chloro(difluoro)methoxy]phenyl]-6-[(3R)-3-hydroxypyrrolidin-1-yl]-5-[2-(2-trimethylsilylethoxymethyl)pyrazol-3-yl]pyridine-3-carboxamide |
InChI |
InChI=1S/C26H32ClF2N5O4Si/c1-39(2,3)13-12-37-17-34-23(8-10-31-34)22-14-18(15-30-24(22)33-11-9-20(35)16-33)25(36)32-19-4-6-21(7-5-19)38-26(27,28)29/h4-8,10,14-15,20,35H,9,11-13,16-17H2,1-3H3,(H,32,36)/t20-/m1/s1 |
InChI Key |
AKTBREKFPHQEON-HXUWFJFHSA-N |
Isomeric SMILES |
C[Si](C)(C)CCOCN1C(=CC=N1)C2=C(N=CC(=C2)C(=O)NC3=CC=C(C=C3)OC(F)(F)Cl)N4CC[C@H](C4)O |
Canonical SMILES |
C[Si](C)(C)CCOCN1C(=CC=N1)C2=C(N=CC(=C2)C(=O)NC3=CC=C(C=C3)OC(F)(F)Cl)N4CCC(C4)O |
Origin of Product |
United States |
Disclaimer and Information on In-Vitro Research Products
Please be aware that all articles and product information presented on BenchChem are intended solely for informational purposes. The products available for purchase on BenchChem are specifically designed for in-vitro studies, which are conducted outside of living organisms. In-vitro studies, derived from the Latin term "in glass," involve experiments performed in controlled laboratory settings using cells or tissues. It is important to note that these products are not categorized as medicines or drugs, and they have not received approval from the FDA for the prevention, treatment, or cure of any medical condition, ailment, or disease. We must emphasize that any form of bodily introduction of these products into humans or animals is strictly prohibited by law. It is essential to adhere to these guidelines to ensure compliance with legal and ethical standards in research and experimentation.