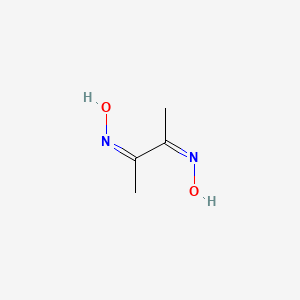
Glyoxime, dimethyl-
- Click on QUICK INQUIRY to receive a quote from our team of experts.
- With the quality product at a COMPETITIVE price, you can focus more on your research.
Overview
Description
Dimethylglyoxime is a chemical compound with the molecular formula C₄H₈N₂O₂. It is a white, crystalline powder that is soluble in methanol and sodium hydroxide solution but insoluble in water . Dimethylglyoxime is widely used in analytical chemistry, particularly for the detection and analysis of nickel and other metals . Its unique chemical properties make it an invaluable tool for chemists and researchers .
Preparation Methods
Dimethylglyoxime can be synthesized from diacetyl monoxime, which is obtained by the reaction of diacetyl with hydroxylamine . The key transformation involves treating diacetyl monoxime with an additional quantity of hydroxylamine in the presence of an acidic catalyst . This reaction leads to the formation of dimethylglyoxime in a crystalline form, which can be purified by recrystallization from hot water . Industrial production methods typically follow similar synthetic routes but on a larger scale .
Chemical Reactions Analysis
Dimethylglyoxime undergoes several types of chemical reactions, including complexation, precipitation, and substitution reactions . One of the most notable reactions is its ability to form a red precipitate with nickel ions, which is used for the qualitative and quantitative determination of nickel . The reaction can be represented as follows: [ \text{Ni}^{2+} + 2\text{C}_4\text{H}_8\text{N}_2\text{O}_2 \rightarrow \text{Ni}(\text{C}_4\text{H}_7\text{N}_2\text{O}_2)_2 \downarrow (\text{red precipitate}) + 2\text{H}^+ ] Dimethylglyoxime also reacts with ferrous sulfate and ammonium hydroxide to form a complex compound of iron and ammonium sulfate .
Scientific Research Applications
Dimethylglyoxime has a wide range of applications in scientific research. In analytical chemistry, it is used as a selective precipitating reagent, detecting reagent, and photometric reagent for nickel, palladium, platinum, and other metal ions . It is also used in the separation of palladium from tin, gold, rhodium, and iridium . In organic synthesis, dimethylglyoxime serves as an efficient ligand for copper-catalyzed hydroxylation of aryl halides . Additionally, it is used in the preparation of hybrid catalysts for the synthesis of various organic compounds .
Mechanism of Action
The mechanism of action of dimethylglyoxime involves its ability to form stable complexes with metal ions . The compound acts as a chelating agent, binding to metal ions through its nitrogen and oxygen atoms . This complexation process is highly specific and selective, allowing for the precise detection and quantification of metal ions in various samples . The formation of these complexes can also facilitate the separation and purification of metals from mixtures .
Comparison with Similar Compounds
Dimethylglyoxime is unique in its high specificity for nickel ions, which sets it apart from other similar compounds . Some related compounds include hydroxylamine and salicylaldoxime, which also form complexes with metal ions but lack the same level of specificity and stability as dimethylglyoxime . Other diketones, such as benzil, can also be used to prepare related ligands, but they do not offer the same versatility and effectiveness in analytical applications .
Properties
CAS No. |
464921-38-8 |
---|---|
Molecular Formula |
C4H8N2O2 |
Molecular Weight |
116.12 g/mol |
IUPAC Name |
(NZ)-N-[(3Z)-3-hydroxyiminobutan-2-ylidene]hydroxylamine |
InChI |
InChI=1S/C4H8N2O2/c1-3(5-7)4(2)6-8/h7-8H,1-2H3/b5-3-,6-4- |
InChI Key |
JGUQDUKBUKFFRO-GLIMQPGKSA-N |
Isomeric SMILES |
C/C(=N/O)/C(=N\O)/C |
Canonical SMILES |
CC(=NO)C(=NO)C |
physical_description |
Crystals soluble in ethanol and slightly soluble in water; [Sax] White powder; [MSDSonline] |
vapor_pressure |
0.00069 [mmHg] |
Origin of Product |
United States |
Disclaimer and Information on In-Vitro Research Products
Please be aware that all articles and product information presented on BenchChem are intended solely for informational purposes. The products available for purchase on BenchChem are specifically designed for in-vitro studies, which are conducted outside of living organisms. In-vitro studies, derived from the Latin term "in glass," involve experiments performed in controlled laboratory settings using cells or tissues. It is important to note that these products are not categorized as medicines or drugs, and they have not received approval from the FDA for the prevention, treatment, or cure of any medical condition, ailment, or disease. We must emphasize that any form of bodily introduction of these products into humans or animals is strictly prohibited by law. It is essential to adhere to these guidelines to ensure compliance with legal and ethical standards in research and experimentation.