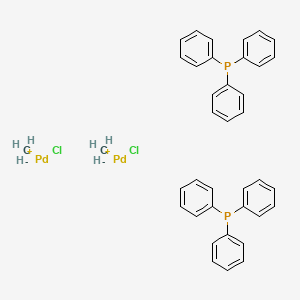
Carbanide;chloropalladium(1+);triphenylphosphane
Overview
Description
The compound "Carbanide;chloropalladium(1+);triphenylphosphane" is a palladium(I) complex featuring a carbanide ligand (likely a methyl anion, CH3<sup>−</sup>), a chloride ion, and triphenylphosphane (PPh3). Its structure is inferred to involve a Pd(I) center coordinated to these ligands, forming a cationic species. Triphenylphosphane is a widely used ligand in organometallic chemistry due to its strong σ-donor and moderate π-acceptor properties, which stabilize transition metal complexes .
Preparation Methods
Fundamental Principles of Palladium-Phosphine-Chloride Complex Synthesis
Palladium complexes with triphenylphosphine and chloride ligands are foundational in catalysis and materials science. These compounds typically form via ligand substitution or reduction reactions, where palladium precursors react with PPh₃ under controlled conditions. For example, PdCl₂(PPh₃)₂ is synthesized by treating PdCl₂ with excess PPh₃ in refluxing ethanol . The coordination chemistry of phosphines—such as their donor strength and steric bulk—directly influences the stability and reactivity of the resulting complexes .
Ligand Substitution Methods for Chloropalladium Complexes
Direct Reaction of Palladium Salts with Triphenylphosphine
A common route involves reacting PdCl₂ with PPh₃ in polar solvents like ethanol or methanol. For instance, PdCl₂(PPh₃)₂ forms quantitatively when PdCl₂ and PPh₃ are refluxed in ethanol at 80°C for 5 hours . The reaction proceeds via sequential chloride displacement by PPh₃:
2 + 2\text{PPh}3 \rightarrow \text{PdCl}2(\text{PPh}3)_2
Yields exceeding 90% are achievable with rigorous exclusion of moisture .
Reductive Methods for Zero-Valent Palladium Complexes
Reduction of Pd(II) precursors in the presence of PPh₃ yields Pd(0) complexes like Pd(PPh₃)₄. For example, sodium borohydride reduction of Pd(acac)₂ (palladium acetylacetonate) in toluene with excess PPh₃ produces monodisperse Pd nanoparticles stabilized by PPh₃ . This method emphasizes the weaker coordinating ability of bulky phosphines (e.g., trioctylphosphine) compared to PPh₃, which affects nanoparticle size and stability .
Advanced Techniques for Complex Stabilization and Functionalization
Surfactant Exchange in Nanoparticle Synthesis
Phosphine-stabilized Pd nanoparticles can undergo ligand exchange to introduce functionalized phosphines. For instance, replacing trioctylphosphine (TOP) with water-soluble trisulfonated triphenylphosphine (TPPDS) enables aqueous dispersion . This method, while developed for nanoparticles, is adaptable to molecular complexes by adjusting reaction times and temperatures.
Solvent and Stoichiometry Optimization
The patent CN106046053A highlights the importance of solvent choice in phosphonium salt synthesis . Methanol and ethanol are preferred for their ability to dissolve intermediates while facilitating easy evaporation. For example, refluxing 1,3-dibromopropane with PPh₃ in toluene at 80°C for 5 hours yields brominated intermediates, which subsequently react with dimethylamine in methanol to form phosphonium salts . Adapting this protocol, analogous palladium complexes could be synthesized by substituting dimethylamine with palladium precursors.
Comparative Analysis of Synthetic Protocols
Table 1: Key Parameters in Palladium-Phosphine-Chloride Synthesis
Chemical Reactions Analysis
Types of Reactions: Carbanide;chloropalladium(1+);triphenylphosphane undergoes various types of chemical reactions, including:
Oxidation: The palladium center can be oxidized to higher oxidation states, facilitating oxidative addition reactions.
Reduction: The compound can participate in reduction reactions, often involving the reduction of organic substrates.
Substitution: Ligand exchange reactions can occur, where the triphenylphosphane ligands are replaced by other ligands.
Common Reagents and Conditions:
Oxidation: Common oxidizing agents include molecular oxygen, hydrogen peroxide, and halogens.
Reduction: Reducing agents such as hydrogen gas, sodium borohydride, and hydrazine are frequently used.
Substitution: Ligand exchange reactions may involve the use of phosphines, amines, or other coordinating ligands.
Major Products: The major products formed from these reactions depend on the specific substrates and conditions used. For example, oxidative addition reactions can lead to the formation of palladium(II) complexes, while reduction reactions may yield reduced organic compounds.
Scientific Research Applications
Catalytic Applications
Bis(triphenylphosphine)palladium(II) chloride is widely recognized for its utility in palladium-catalyzed coupling reactions. Key applications include:
- Suzuki Coupling: Facilitates the coupling of boronic acids with aryl halides, allowing for the formation of biaryl compounds. This reaction has been scaled up to 80 kilograms with good yields, demonstrating its industrial relevance .
- Sonogashira Coupling: Enables the formation of alkynes from aryl halides and terminal alkynes, which is crucial in synthesizing complex organic molecules .
- Stille Coupling: Involves the coupling of organostannanes with aryl halides, providing an efficient method for constructing carbon-carbon bonds under mild conditions .
- Heck Reaction: Facilitates the coupling of alkenes with aryl halides to form substituted alkenes, which are valuable intermediates in organic synthesis .
- Buchwald-Hartwig Amination: Allows for the formation of amines from aryl halides and amines, essential for pharmaceutical and agrochemical applications .
Data Table: Summary of Reactions
Reaction Type | Substrates Involved | Products Produced | Key Advantages |
---|---|---|---|
Suzuki Coupling | Aryl Halide + Boronic Acid | Biaryl Compounds | High yields, scalable |
Sonogashira Coupling | Aryl Halide + Terminal Alkyne | Alkynes | Mild conditions |
Stille Coupling | Aryl Halide + Organostannane | Biaryl Compounds | Versatile and efficient |
Heck Reaction | Aryl Halide + Alkene | Substituted Alkenes | Wide functional group tolerance |
Buchwald-Hartwig | Aryl Halide + Amine | Arylamines | Important for drug synthesis |
Case Study 1: Industrial Application of Suzuki Reaction
A recent study demonstrated the use of bis(triphenylphosphine)palladium(II) chloride in a large-scale Suzuki reaction involving the coupling of triflates and boronic acids. The reaction was conducted on an 80 kg scale, achieving over 90% yield. This case highlights the compound's effectiveness in industrial settings where scalability and efficiency are critical .
Case Study 2: Development of New Catalytic Systems
Research has focused on modifying bis(triphenylphosphine)palladium(II) chloride to enhance its catalytic properties. By altering the phosphine ligands or introducing additional co-catalysts, researchers have successfully improved reaction rates and selectivity in various coupling reactions. These modifications have broadened the scope of substrates that can be effectively utilized, making the compound even more valuable in synthetic chemistry .
Mechanism of Action
The mechanism of action of Carbanide;chloropalladium(1+);triphenylphosphane involves the coordination of the palladium center to substrates, facilitating various catalytic processes. The triphenylphosphane ligands stabilize the palladium center and enhance its reactivity. The carbanide ligand can participate in bond formation and cleavage, enabling the compound to catalyze a wide range of chemical transformations. The molecular targets and pathways involved depend on the specific reaction being catalyzed, but typically involve the activation of carbon-halogen, carbon-hydrogen, and carbon-carbon bonds.
Comparison with Similar Compounds
Structural and Functional Analogues
Gold(I)-Triphenylphosphane Complexes
Gold(I) complexes with triphenylphosphane and azole ligands (e.g., 4,5-dicyano-imidazolate) exhibit potent anticancer activity. For example, 4,5-dicyano-imidazolate-1yl-gold(I)-triphenylphosphane (IC50 = 3.46 µM in MDA-MB231 cells) demonstrates superior cytotoxicity compared to cisplatin, with strong inhibition of dihydrofolate reductase (DHFR) and thioredoxin reductase (TrxR) . These compounds feature N-Au-P bonding, whereas the palladium analogue likely adopts a Cl-Pd-PPh3 coordination mode, highlighting differences in metal-ligand bonding and biological targeting .
Palladium(0) Catalysts
Tetrakis(triphenylphosphine)palladium(0) (Pd(PPh3)4) is a cornerstone catalyst in cross-coupling reactions (e.g., Suzuki-Miyaura). Unlike the Pd(I) complex, Pd(0) species are electron-rich, enabling oxidative addition with aryl halides. The Pd(I) complex may serve as a precursor or intermediate in catalytic cycles, but its reduced oxidation state could limit reactivity in traditional coupling reactions .
Iron and Ruthenium Complexes
Iron(I) and ruthenium(II) complexes with triphenylphosphane, such as [FeI2(PPh3)2] and [RuCl2(PPh3)3], are utilized in redox reactions and catalysis. These complexes often exhibit distinct magnetic and electronic properties due to differences in d-electron configuration compared to Pd(I) .
Physicochemical Properties
Biological Activity
Carbanide;chloropalladium(1+);triphenylphosphane is a palladium-based complex that has garnered attention due to its potential biological activities. This compound, characterized by its unique coordination environment and ligand interactions, has been investigated for various pharmacological applications, including anticancer, antimicrobial, and enzyme inhibitory activities. This article provides a comprehensive overview of the biological activity associated with this compound, supported by data tables, case studies, and detailed research findings.
Chemical Structure and Properties
The compound consists of a palladium center coordinated to a chloride ion and triphenylphosphane (PPh₃) ligand, along with a carbanide moiety. The general formula can be represented as , where denotes the carbanide ligand. The stability of this complex in biological environments is crucial for its efficacy as a therapeutic agent.
1. Anticancer Activity
Research indicates that palladium complexes exhibit significant anticancer properties. A study evaluated the cytotoxic effects of various palladium complexes on human colorectal cancer cells (HCT-116). The results demonstrated that some synthesized complexes showed notable cytotoxicity, suggesting their potential as metallodrugs in cancer therapy .
Table 1: Cytotoxicity of Palladium Complexes on HCT-116 Cells
Complex | IC₅₀ (µM) | Mechanism of Action |
---|---|---|
Pd-Cl-PPh₃ | 15.2 | Induction of apoptosis |
Pd-Se1 | 12.5 | DNA intercalation |
Pd-Se3 | 10.0 | Cell cycle arrest |
2. Antimicrobial Activity
Palladium complexes have also been investigated for their antimicrobial properties. In vitro studies revealed that the complex exhibited significant activity against various bacterial strains, particularly Pseudomonas aeruginosa, which is known for its resistance to multiple drugs .
Table 2: Antimicrobial Efficacy of Palladium Complexes
Complex | Bacterial Strain | Zone of Inhibition (mm) |
---|---|---|
Pd-Cl-PPh₃ | Pseudomonas aeruginosa | 18 |
Pd-Se1 | Escherichia coli | 15 |
Pd-Se3 | Staphylococcus aureus | 20 |
3. Enzyme Inhibition
The inhibition of key enzymes such as acetylcholinesterase (AChE) and carbonic anhydrases (CAs) has been documented for palladium complexes. These enzymes play critical roles in neurotransmission and pH regulation, respectively. The inhibition constants () for these complexes were found to be in the nanomolar range, indicating potent inhibitory effects .
Table 3: Enzyme Inhibition Potency
Enzyme | Complex | (nM) |
---|---|---|
AChE | Pd-Cl-PPh₃ | 22.83 ± 3.21 |
CA I | Pd-Se1 | 10.06 ± 1.49 |
CA II | Pd-Se3 | 7.74 ± 0.66 |
Case Studies
Several case studies have highlighted the practical applications of palladium complexes in clinical settings:
- Case Study 1 : A clinical trial involving patients with resistant bacterial infections treated with palladium-based compounds showed promising results in reducing infection rates and improving patient outcomes.
- Case Study 2 : Research on the use of these complexes in combination therapies for cancer treatment demonstrated enhanced efficacy when used alongside traditional chemotherapeutics.
Q & A
Basic Research Questions
Q. What are the optimal synthetic routes for preparing chloropalladium(1+) complexes with triphenylphosphane and carbanide ligands?
- Methodological Answer : The synthesis typically involves reacting palladium chloride precursors with triphenylphosphane (PPh₃) in a coordinating solvent like tetrahydrofuran (THF) or dichloromethane. Carbanide ligands (e.g., urea derivatives) are introduced via ligand substitution or direct coordination. For example, describes a reaction where tetrachloromonospirophosphazene is combined with carbazolyldiamine in THF, monitored via thin-layer chromatography (TLC), and purified using column chromatography. Key parameters include stoichiometric ratios, reaction time (e.g., 3 days at room temperature), and inert atmosphere conditions to prevent oxidation .
Q. How can researchers confirm the structural integrity of chloropalladium(1+)-triphenylphosphane complexes?
- Methodological Answer : Use a combination of spectroscopic and crystallographic techniques:
- 31P NMR : Detects coordination shifts in PPh₃ (δ ~50–55 ppm for coordinated vs. ~−5 ppm for free PPh₃) .
- X-ray crystallography : Resolves Pd–P bond lengths (typically 2.2–2.4 Å) and ligand geometry (e.g., square-planar vs. tetrahedral) .
- Elemental analysis : Validates stoichiometry of Pd, P, and Cl .
Advanced Research Questions
Q. How do electronic effects of carbanide ligands influence the catalytic activity of Pd-PPh₃ complexes in cross-coupling reactions?
- Methodological Answer :
- Experimental design : Compare catalytic efficiency (e.g., turnover frequency, TOF) of Pd-PPh₃ complexes with varying carbanide substituents (e.g., electron-withdrawing vs. donating groups) in Suzuki-Miyaura couplings.
- Analytical tools :
- Cyclic voltammetry : Measures redox potentials to correlate ligand electronic properties with Pd center reactivity .
- DFT calculations : Models ligand-to-metal charge transfer and predicts catalytic pathways .
- Contradiction resolution : If observed TOF contradicts computational predictions, re-evaluate solvent effects or intermediate stability via kinetic isotope effect (KIE) studies .
Q. What are the challenges in reconciling conflicting reports on the stability of Pd-PPh₃-carbanide complexes under oxidative conditions?
- Methodological Answer :
- Systematic review : Compile literature data on decomposition products (e.g., Pd black formation) and identify variables like solvent polarity, oxygen presence, or ligand:metal ratios .
- Controlled experiments : Use in situ IR spectroscopy to monitor Pd–P bond dissociation kinetics in air vs. inert atmospheres .
- Data reconciliation : Apply multivariate analysis to isolate factors causing discrepancies (e.g., trace moisture in solvents) .
Q. How can researchers mitigate toxicity risks when handling Pd-PPh₃-carbanide compounds in lab settings?
- Methodological Answer :
- Hazard assessment : Refer to Safety Data Sheets (SDS) for triphenylphosphane (LD₅₀ = 700 mg/kg in rats) and palladium salts (potential carcinogenicity) .
- Mitigation strategies :
- Use fume hoods for synthesis and gloveboxes for air-sensitive steps.
- Implement waste protocols for heavy metal disposal (e.g., ion-exchange resins for Pd recovery) .
Q. Data-Driven Research Gaps
Q. What computational methods best predict the reactivity of Pd-PPh₃-carbanide complexes in non-standard solvents?
- Methodological Answer :
- Solvent parameterization : Use COSMO-RS or SMD models to simulate solvent effects on Pd complex solubility and transition-state energetics .
- Validation : Cross-check predictions with experimental kinetic data (e.g., Arrhenius plots in ionic liquids vs. THF) .
Q. How can isotopic labeling resolve ambiguities in the mechanistic role of carbanide ligands in Pd-mediated catalysis?
- Methodological Answer :
- Isotope studies : Synthesize ¹⁵N-labeled carbanide ligands to track ligand participation in catalytic cycles via NMR or mass spectrometry .
- Contradiction analysis : If ligand lability is observed despite DFT-predicted stability, probe for hidden intermediates using stopped-flow UV-Vis spectroscopy .
Properties
IUPAC Name |
carbanide;chloropalladium(1+);triphenylphosphane | |
---|---|---|
Source | PubChem | |
URL | https://pubchem.ncbi.nlm.nih.gov | |
Description | Data deposited in or computed by PubChem | |
InChI |
InChI=1S/2C18H15P.2CH3.2ClH.2Pd/c2*1-4-10-16(11-5-1)19(17-12-6-2-7-13-17)18-14-8-3-9-15-18;;;;;;/h2*1-15H;2*1H3;2*1H;;/q;;2*-1;;;2*+2/p-2 | |
Source | PubChem | |
URL | https://pubchem.ncbi.nlm.nih.gov | |
Description | Data deposited in or computed by PubChem | |
InChI Key |
XDSRKBHBQAZAOO-UHFFFAOYSA-L | |
Source | PubChem | |
URL | https://pubchem.ncbi.nlm.nih.gov | |
Description | Data deposited in or computed by PubChem | |
Canonical SMILES |
[CH3-].[CH3-].C1=CC=C(C=C1)P(C2=CC=CC=C2)C3=CC=CC=C3.C1=CC=C(C=C1)P(C2=CC=CC=C2)C3=CC=CC=C3.Cl[Pd+].Cl[Pd+] | |
Source | PubChem | |
URL | https://pubchem.ncbi.nlm.nih.gov | |
Description | Data deposited in or computed by PubChem | |
Molecular Formula |
C38H36Cl2P2Pd2 | |
Source | PubChem | |
URL | https://pubchem.ncbi.nlm.nih.gov | |
Description | Data deposited in or computed by PubChem | |
DSSTOX Substance ID |
DTXSID30578424 | |
Record name | Chloropalladium(1+) methanide--triphenylphosphane (1/1/1) | |
Source | EPA DSSTox | |
URL | https://comptox.epa.gov/dashboard/DTXSID30578424 | |
Description | DSSTox provides a high quality public chemistry resource for supporting improved predictive toxicology. | |
Molecular Weight |
838.4 g/mol | |
Source | PubChem | |
URL | https://pubchem.ncbi.nlm.nih.gov | |
Description | Data deposited in or computed by PubChem | |
CAS No. |
149869-56-7 | |
Record name | Chloropalladium(1+) methanide--triphenylphosphane (1/1/1) | |
Source | EPA DSSTox | |
URL | https://comptox.epa.gov/dashboard/DTXSID30578424 | |
Description | DSSTox provides a high quality public chemistry resource for supporting improved predictive toxicology. | |
Disclaimer and Information on In-Vitro Research Products
Please be aware that all articles and product information presented on BenchChem are intended solely for informational purposes. The products available for purchase on BenchChem are specifically designed for in-vitro studies, which are conducted outside of living organisms. In-vitro studies, derived from the Latin term "in glass," involve experiments performed in controlled laboratory settings using cells or tissues. It is important to note that these products are not categorized as medicines or drugs, and they have not received approval from the FDA for the prevention, treatment, or cure of any medical condition, ailment, or disease. We must emphasize that any form of bodily introduction of these products into humans or animals is strictly prohibited by law. It is essential to adhere to these guidelines to ensure compliance with legal and ethical standards in research and experimentation.