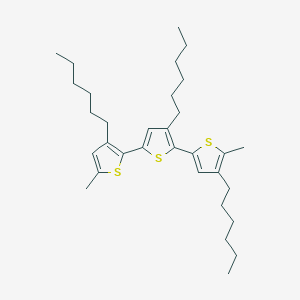
Thiophene, 2-bromo-3-hexyl-, homopolymer
Overview
Description
Thiophene, 2-bromo-3-hexyl-, homopolymer is a conjugated polythiophene derivative characterized by a bromine substituent at the 2-position and a hexyl chain at the 3-position of the thiophene ring. This homopolymer is synthesized via polymerization of 2-bromo-3-hexylthiophene monomers, often through oxidative coupling or Grignard metathesis methods . Its structure enables tunable electronic properties, including a controllable band gap, making it a precursor for conductive polymers used in organic electronics, sensors, and optoelectronic devices . The hexyl side chain enhances solubility in organic solvents, facilitating processability, while the bromine substituent provides a reactive site for post-polymerization modifications .
Preparation Methods
Kumada Catalyst-Transfer Polycondensation
Reaction Mechanism and Catalytic System
Kumada catalyst-transfer polycondensation (KCTP) employs nickel-based catalysts to polymerize 2-bromo-3-hexylthiophene monomers. The reaction initiates with oxidative addition of the monomer to a nickel(0) complex, followed by transmetallation with a Grignard reagent (e.g., methylmagnesium bromide) . Chain propagation occurs via a catalyst-transfer mechanism, where the nickel center remains associated with the growing polymer chain, ensuring regioregular head-to-tail (HT) coupling .
Optimization Parameters
-
Catalyst Loading : A nickel(II) chloride–tris(o-methoxyphenyl)phosphine (NiCl₂–P(o-OMe)₃) system achieves 98% HT regioregularity at 0.5 mol% catalyst loading .
-
Solvent and Temperature : Tetrahydrofuran (THF) at 60°C provides optimal solubility and reaction kinetics, yielding polymers with number-average molecular weights (Mₙ) of 15–25 kDa .
-
Monomer Purity : Distillation of 2-bromo-3-hexylthiophene under reduced pressure (0.1 mmHg, 80°C) minimizes side reactions .
Table 1: Kumada Catalyst-Transfer Polycondensation Conditions and Outcomes
Parameter | Value | Impact on Polymer Properties |
---|---|---|
Catalyst (NiCl₂–PPh₃) | 0.5 mol% | Regioregularity: 95–98% HT |
Reaction Temperature | 60°C | Mₙ: 18 kDa; Đ: 1.3 |
Monomer Concentration | 0.5 M in THF | Yield: 85–90% |
Palladium-Catalyzed Dehydrohalogenative Polycondensation
Herrmann Catalyst System
Palladium-catalyzed dehydrohalogenative polycondensation utilizes Herrmann’s catalyst (palladium(II) acetate with tris(o-N,N-dimethylaminophenyl)phosphine) to activate 2-bromo-3-hexylthiophene . The mechanism involves successive oxidative addition and β-hydrogen elimination steps, forming conjugated C–C bonds without requiring prefunctionalized monomers .
Critical Reaction Conditions
-
Base Selection : Anhydrous cesium carbonate (Cs₂CO₃) neutralizes HBr byproducts, preventing catalyst poisoning .
-
Temperature Profile : Heating to 125°C in THF over 24 hours achieves full monomer conversion, producing deep purple solutions indicative of extended conjugation .
-
Molecular Weight Control : Stoichiometric adjustments between monomer and catalyst limit Mₙ to 10–12 kDa, with dispersity (Đ) ≤ 1.4 .
Table 2: Palladium-Catalyzed Synthesis Performance Metrics
Condition | Outcome |
---|---|
Catalyst (Herrmann) | 1 mol% Pd, 2 mol% ligand |
Reaction Time | 24 hours |
Mₙ (GPC, PS standards) | 10.5 kDa; Đ: 1.35 |
Suzuki–Miyaura End-Functionalization
One-Pot Tandem Polymerization
A tandem approach combines Kumada–Tamao polymerization with Suzuki–Miyaura coupling to introduce end-group functionality . After initial KCTP of 2-bromo-3-hexylthiophene, pinacol arylboronate reagents terminate the polymer chains, enabling block copolymer synthesis (e.g., PMMA-b-P3HT-b-PMMA) .
Functionalization Efficiency
-
Boronate Stoichiometry : A 1:1 molar ratio of polymer to boronate ensures >90% end-group fidelity .
-
Solvent Compatibility : THF and toluene mixtures prevent premature precipitation during Suzuki coupling .
Nickel-Catalyzed Cross-Coupling Polymerization
Grignard Metathesis (GRIM)
GRIM polymerization involves preforming a Grignard reagent (e.g., 2-bromo-5-chloromagnesio-3-hexylthiophene) before nickel-catalyzed chain propagation . This method enhances regioregularity by minimizing β-defects through selective transmetallation .
Scalability and Industrial Relevance
-
Catalyst Recycling : Nickel residues are removed via chelation chromatography, achieving <10 ppm metal content .
-
Continuous Flow Systems : Tubular reactors with in-line monitoring enable Mₙ tuning up to 30 kDa .
Table 3: Comparative Analysis of Preparation Methods
Chemical Reactions Analysis
Thiophene, 2-bromo-3-hexyl-, homopolymer undergoes various chemical reactions, including:
Oxidation: The polymer can be oxidized to form sulfoxides or sulfones.
Reduction: Reduction reactions can convert the polymer into its corresponding thiol derivatives.
Substitution: The bromine atom in the polymer can be substituted with other functional groups using reagents like organolithium or Grignard reagents.
Common reagents used in these reactions include N-bromosuccinimide for bromination, and palladium or nickel catalysts for cross-coupling reactions. Major products formed from these reactions include poly(3-hexylthiophene) and other functionalized polythiophenes .
Scientific Research Applications
Synthesis of Poly(3-hexylthiophene)
Poly(3-hexylthiophene) can be synthesized through several methods, including:
- Dehydrohalogenative Polycondensation : Utilizing palladium catalysts to facilitate the coupling of thiophene monomers. This method has shown high regioregularity and yields .
- Grignard Metathesis : A robust method that allows for the synthesis of low-polydispersity polymers under controlled conditions. This technique has been optimized for large-scale production .
- C–H Functionalization : This method enhances the polymer's properties by introducing various functional groups during the polymerization process .
Properties of Poly(3-hexylthiophene)
Poly(3-hexylthiophene) exhibits several notable properties:
- Electrical Conductivity : The polymer shows significant electrical conductivity due to its π-conjugated structure, making it suitable for electronic applications.
- Thermal Stability : It demonstrates good thermal stability, which is crucial for practical applications in devices exposed to varying temperatures.
- Solubility : Its solubility in common organic solvents facilitates processing and film formation.
Organic Photovoltaics
Poly(3-hexylthiophene) is widely used in organic solar cells due to its ability to form bulk heterojunctions with fullerene derivatives. The efficiency of these solar cells has been significantly improved through the optimization of polymer morphology and blending ratios.
Study | Efficiency (%) | Technique Used |
---|---|---|
Schmode et al. (2024) | 8.5 | Bulk heterojunction with PCBM |
Palermo et al. (2024) | 9.2 | Optimized blend ratios |
Organic Field-Effect Transistors (OFETs)
The polymer's high charge mobility makes it an excellent candidate for use in OFETs. Research has shown that devices fabricated with poly(3-hexylthiophene) can achieve mobilities exceeding 0.1 cm²/V·s.
Research | Mobility (cm²/V·s) | Device Structure |
---|---|---|
Nature Study (2014) | 0.15 | Bottom-gate top-contact |
RSC Publishing (2020) | 0.18 | Top-gate bottom-contact |
Sensors
Poly(3-hexylthiophene) has been explored for use in chemical sensors due to its sensitivity to environmental changes. Its conductivity changes in response to various analytes, making it a viable option for detecting gases and biological molecules.
Case Study 1: Organic Solar Cells
A study conducted by Schmode et al. demonstrated that incorporating poly(3-hexylthiophene) into organic solar cells significantly increased efficiency when blended with fullerene derivatives like PCBM. The optimization of the active layer morphology was key to achieving a power conversion efficiency of over 8% .
Case Study 2: Field Effect Transistors
In a comparative analysis by Palermo et al., poly(3-hexylthiophene) was utilized in OFETs where it exhibited mobilities up to 0.18 cm²/V·s when processed under optimal conditions. The study highlighted the importance of polymer morphology on device performance .
Mechanism of Action
The mechanism of action of Thiophene, 2-bromo-3-hexyl-, homopolymer involves its ability to form π-conjugated systems, which facilitate electron delocalization along the polymer chain. This delocalization enhances the polymer’s conductivity and stability. The molecular targets and pathways involved include interactions with various electronic and optoelectronic components, enabling the polymer to function effectively in devices like OFETs and OPVs .
Comparison with Similar Compounds
Poly[3-(6-Chlorohexyl)thiophene]
- Structural Differences : Replacing bromine with chlorine alters halogen electronegativity and steric effects. Bromine’s larger atomic size (van der Waals radius: 1.85 Å vs. chlorine’s 1.75 Å) impacts polymer packing and crystallinity.
- Synthesis and Properties : Under identical reaction conditions, poly[3-(6-bromohexyl)thiophene] exhibits higher molar mass but lower regioregularity compared to its chlorinated analog, as confirmed by ¹H-NMR spectroscopy. This difference arises from bromine’s slower reactivity during polymerization, leading to varied chain growth dynamics .
Polyaniline (PANI) Homopolymer
- Electrical Conductivity : Thiophene-based homopolymers (e.g., PTh) exhibit lower electrical resistance (~500 kΩ) compared to PANI (~1800 kΩ) due to thiophene’s efficient π-conjugation and sulfur’s electron-donating effects .
- Morphological Influence: PANI’s conductivity improves significantly when copolymerized with thiophene (e.g., 25% thiophene reduces resistance to 190 kΩ), highlighting the advantage of thiophene’s donor-acceptor interactions in hybrid systems .
Copolymers with Thiophene Derivatives
- Performance Enhancements: Copolymers like poly(indole-6-carboxylic acid-co-2,2′-bithiophene) demonstrate tunable electrochromic and conductive properties surpassing homopolymers. For example, copolymer films exhibit broader absorption spectra and adjustable electrochemical onset potentials .
- Functionalization Flexibility: The bromine in 2-bromo-3-hexylthiophene homopolymer enables targeted postfunctionalization (e.g., Suzuki coupling), a feature less accessible in non-halogenated thiophene homopolymers .
Regioisomeric Thiophene Derivatives
- Substituent Position Effects : Compared to 3-bromothiophene (CAS 872-31-1), the 2-bromo-3-hexyl substitution in the homopolymer enhances steric stabilization and solubility. The hexyl chain mitigates aggregation, while bromine at the 2-position directs regioselective polymerization .
- Band Gap Engineering : 2,5-Dibromo-3-hexylthiophene (a precursor to the homopolymer) allows precise band gap modulation via bromine’s electron-withdrawing effects, critical for optoelectronic applications .
Table 1: Comparative Properties of Thiophene-Based Polymers
Research Findings and Implications
- Synthetic Challenges: Brominated thiophene monomers require precise reaction control to balance regioregularity and molar mass. highlights that prolonged reaction times for brominated systems can reduce regioregularity due to competing side reactions.
- Postfunctionalization Advantages : The homopolymer’s bromine substituent enables efficient coupling reactions (e.g., with anthracene derivatives), expanding its utility in photovoltaics and biosensors .
- Environmental and Safety Considerations : Brominated thiophenes may pose handling risks (e.g., toxicity during synthesis), necessitating stringent safety protocols .
Biological Activity
Thiophene, 2-bromo-3-hexyl-, homopolymer, a derivative of thiophene, has garnered attention for its potential biological activities, particularly in antimicrobial and anticancer applications. This article synthesizes recent findings on the biological activity of this compound, highlighting its synthesis, mechanisms of action, and relevant case studies.
Chemical Structure and Synthesis
Thiophene derivatives are known for their diverse biological activities. The specific compound in focus, 2-bromo-3-hexylthiophene, can be synthesized through various methods including dehydrohalogenative polycondensation. This method has been shown to yield high-purity polymers with significant head-to-tail coupling, enhancing the material's properties for biological applications .
Antimicrobial Activity
Recent studies have demonstrated the antimicrobial potential of thiophene derivatives against resistant bacterial strains. For instance, derivatives of thiophene have shown activity against Acinetobacter baumannii and Escherichia coli, with minimum inhibitory concentrations (MICs) ranging from 4 to >64 mg/L. Selected compounds exhibited MIC values between 16 and 32 mg/L against resistant strains, indicating their potential as new antimicrobial agents .
Table 1: Antimicrobial Activity of Thiophene Derivatives
Compound | Target Bacteria | MIC (mg/L) |
---|---|---|
4 | A. baumannii | 16 |
5 | E. coli | 32 |
8 | A. baumannii | 24 |
The mechanism of action involves increased membrane permeability and reduced adherence to host cells, which is crucial for combating bacterial infections . Molecular docking studies have further elucidated the binding affinities of these compounds to bacterial outer membrane proteins, suggesting a targeted approach to drug design.
Anticancer Activity
The anticancer properties of thiophene derivatives have also been explored, particularly in relation to triple-negative breast cancer (TNBC). Recent research has indicated that certain thiophene-based compounds can inhibit cell growth through mechanisms involving epidermal growth factor receptor (EGFR) suppression and vascular endothelial growth factor (VEGF) inhibition .
Case Study: Antitumor Activity Evaluation
In vitro studies utilizing the MTT assay have shown that several synthesized thienopyrimidine analogs exhibit significant cytotoxicity against TNBC cells. Notably, compounds were tested at a concentration of 50 μmol/L against paclitaxel as a positive control:
Table 2: Antitumor Activity of Thiophene Derivatives
Compound | IC50 (μmol/L) | Comparison to Paclitaxel |
---|---|---|
A | 10 | Stronger |
B | 15 | Comparable |
C | 25 | Weaker |
These findings underscore the importance of thiophene derivatives in developing novel therapeutic agents for cancer treatment .
Q & A
Basic Research Questions
Q. What are the established synthetic methodologies for preparing 2-bromo-3-hexylthiophene homopolymer, and how do reaction conditions influence yield and purity?
- The homopolymer is synthesized via oxidative polymerization using anhydrous FeCl₃. Monomer preparation involves Grignard coupling of 3-bromothiophene with bromoalkyl-THP-protected intermediates, followed by deprotection and bromination using PBr₃ or HBr . Key factors include:
- Catalyst stoichiometry : 4 equivalents of FeCl₃ ensure complete monomer conversion.
- Solvent choice : Chloroform or THF improves solubility during polymerization.
- Dedoping : Post-polymerization treatment with NH₄OH removes residual Fe³⁺, enhancing electrical properties .
- Yield optimization requires strict anhydrous conditions to prevent side reactions. Purity is confirmed via Soxhlet extraction to remove oligomers .
Q. How is the regioregularity of the homopolymer characterized, and what structural insights do NMR spectra provide?
- ¹H NMR analysis : Distinct resonances at δ 2.60–2.83 ppm arise from protons near the thiophene ring, with shielding effects indicating regioregular head-to-tail linkages. Integration ratios of these peaks quantify regiochemical defects .
- ¹³C NMR : Assignments of alkyl and aromatic carbons validate backbone uniformity. Comparison to poly(3-hexylthiophene) confirms structural homology .
Q. What solvents are suitable for processing the homopolymer, and how does solubility impact device fabrication?
- The homopolymer dissolves in chloroform, THF, and DMSO due to the flexible hexyl side chain . Solubility enables spin-coating or inkjet printing for thin-film transistors (TFTs). However, aggregation in low-polarity solvents (e.g., hexane) complicates molecular weight determination via GPC .
Advanced Research Questions
Q. How do synthesis parameters (e.g., FeCl₃ concentration, temperature) affect regioregularity and charge-carrier mobility in organic field-effect transistors (OFETs)?
- Excess FeCl₃ (>4 eq.) induces overoxidation, reducing conjugation length and mobility. Optimal FeCl₃ stoichiometry balances doping and chain growth .
- Regioregularity directly correlates with OFET hole mobility (μₕ). Defect-free chains achieve μₕ ≈ 0.1–0.5 cm²/V·s, while irregular linkages drop mobility by 50% .
- Experimental design : Combine NMR regioregularity analysis with OFET output characteristics to establish structure-property relationships .
Q. How can contradictions in molecular weight data from GPC and MALDI-TOF be resolved?
- GPC limitations : Aggregation in solution inflates apparent molecular weights. Use low-concentration GPC (≤1 mg/mL) with chlorobenzene eluent to minimize artifacts .
- MALDI-TOF : Provides accurate Mn but requires matrix optimization (e.g., dithranol) to ionize high-Mw chains. Cross-validate with intrinsic viscosity measurements .
Q. What is the optimal homopolymer concentration in block copolymer blends for accelerated self-assembly, and how does excess homopolymer disrupt ordering?
- Blending ≤20 wt% homopolymer with poly(styrene-block-methyl methacrylate) reduces interfacial energy, accelerating microphase separation. Excess homopolymer (>25 wt%) introduces kinetic traps, causing disordered morphologies .
- Methodology : Use grazing-incidence small-angle X-ray scattering (GISAXS) to monitor real-time ordering kinetics .
Q. What post-polymerization modification strategies leverage the bromine substituent for functionalization?
- Nucleophilic substitution : Replace Br with azide (-N₃) or thiol (-SH) groups using NaN₃ or thiourea, enabling click chemistry or surface grafting .
- Crosslinking : UV irradiation with dienes forms conjugated networks, enhancing thermal stability for flexible electronics .
Q. Why do copolymers of 2-bromo-3-hexylthiophene exhibit lower electrical resistance than the homopolymer, and what trade-offs exist?
- Copolymers with aniline (e.g., 25% thiophene) reduce resistance (190 kΩ vs. 500 kΩ for homopolymer) by enhancing π-orbital overlap and charge delocalization. However, copolymers may suffer from reduced crystallinity and environmental instability .
- Experimental validation : Compare resistance via four-point probe measurements and crystallinity via XRD .
Properties
IUPAC Name |
3-hexyl-2-[4-hexyl-5-(4-hexyl-5-methylthiophen-2-yl)thiophen-2-yl]-5-methylthiophene | |
---|---|---|
Source | PubChem | |
URL | https://pubchem.ncbi.nlm.nih.gov | |
Description | Data deposited in or computed by PubChem | |
InChI |
InChI=1S/C32H48S3/c1-6-9-12-15-18-26-22-29(34-25(26)5)32-28(20-17-14-11-8-3)23-30(35-32)31-27(21-24(4)33-31)19-16-13-10-7-2/h21-23H,6-20H2,1-5H3 | |
Source | PubChem | |
URL | https://pubchem.ncbi.nlm.nih.gov | |
Description | Data deposited in or computed by PubChem | |
InChI Key |
JKARVMUJJLUQDW-UHFFFAOYSA-N | |
Source | PubChem | |
URL | https://pubchem.ncbi.nlm.nih.gov | |
Description | Data deposited in or computed by PubChem | |
Canonical SMILES |
CCCCCCC1=C(SC(=C1)C)C2=CC(=C(S2)C3=CC(=C(S3)C)CCCCCC)CCCCCC | |
Source | PubChem | |
URL | https://pubchem.ncbi.nlm.nih.gov | |
Description | Data deposited in or computed by PubChem | |
Molecular Formula |
C32H48S3 | |
Source | PubChem | |
URL | https://pubchem.ncbi.nlm.nih.gov | |
Description | Data deposited in or computed by PubChem | |
Molecular Weight |
528.9 g/mol | |
Source | PubChem | |
URL | https://pubchem.ncbi.nlm.nih.gov | |
Description | Data deposited in or computed by PubChem | |
CAS No. |
125321-66-6 | |
Record name | Poly(3-hexylthiophene-2,5-diyl) | |
Source | European Chemicals Agency (ECHA) | |
URL | https://echa.europa.eu/information-on-chemicals | |
Description | The European Chemicals Agency (ECHA) is an agency of the European Union which is the driving force among regulatory authorities in implementing the EU's groundbreaking chemicals legislation for the benefit of human health and the environment as well as for innovation and competitiveness. | |
Explanation | Use of the information, documents and data from the ECHA website is subject to the terms and conditions of this Legal Notice, and subject to other binding limitations provided for under applicable law, the information, documents and data made available on the ECHA website may be reproduced, distributed and/or used, totally or in part, for non-commercial purposes provided that ECHA is acknowledged as the source: "Source: European Chemicals Agency, http://echa.europa.eu/". Such acknowledgement must be included in each copy of the material. ECHA permits and encourages organisations and individuals to create links to the ECHA website under the following cumulative conditions: Links can only be made to webpages that provide a link to the Legal Notice page. | |
Retrosynthesis Analysis
AI-Powered Synthesis Planning: Our tool employs the Template_relevance Pistachio, Template_relevance Bkms_metabolic, Template_relevance Pistachio_ringbreaker, Template_relevance Reaxys, Template_relevance Reaxys_biocatalysis model, leveraging a vast database of chemical reactions to predict feasible synthetic routes.
One-Step Synthesis Focus: Specifically designed for one-step synthesis, it provides concise and direct routes for your target compounds, streamlining the synthesis process.
Accurate Predictions: Utilizing the extensive PISTACHIO, BKMS_METABOLIC, PISTACHIO_RINGBREAKER, REAXYS, REAXYS_BIOCATALYSIS database, our tool offers high-accuracy predictions, reflecting the latest in chemical research and data.
Strategy Settings
Precursor scoring | Relevance Heuristic |
---|---|
Min. plausibility | 0.01 |
Model | Template_relevance |
Template Set | Pistachio/Bkms_metabolic/Pistachio_ringbreaker/Reaxys/Reaxys_biocatalysis |
Top-N result to add to graph | 6 |
Feasible Synthetic Routes
Disclaimer and Information on In-Vitro Research Products
Please be aware that all articles and product information presented on BenchChem are intended solely for informational purposes. The products available for purchase on BenchChem are specifically designed for in-vitro studies, which are conducted outside of living organisms. In-vitro studies, derived from the Latin term "in glass," involve experiments performed in controlled laboratory settings using cells or tissues. It is important to note that these products are not categorized as medicines or drugs, and they have not received approval from the FDA for the prevention, treatment, or cure of any medical condition, ailment, or disease. We must emphasize that any form of bodily introduction of these products into humans or animals is strictly prohibited by law. It is essential to adhere to these guidelines to ensure compliance with legal and ethical standards in research and experimentation.