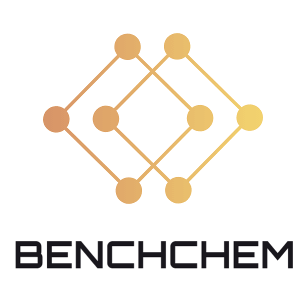
p-NO2-Bn-DOTA(B-199)
- Click on QUICK INQUIRY to receive a quote from our team of experts.
- With the quality product at a COMPETITIVE price, you can focus more on your research.
Overview
Description
P-NO2-Bn-DOTA(B-199) is a novel organometallic compound with a wide range of applications in scientific research. It is a derivative of the molecule 1,4,7,10-tetraazacyclododecane-N,N’,N’’,N’’’-tetraacetic acid (DOTA) and contains a nitro group on the para position of the benzene ring. This compound has been used in a variety of research applications, including magnetic resonance imaging (MRI), radiopharmaceuticals, and chelation therapy.
Scientific Research Applications
Development of Gallium-Based Radiopharmaceuticals
A study conducted by Ferreira et al. (2010) compared different BFCs, including p-NO2-Bn-DOTA, for their efficiency in radiolabeling with gallium radioisotopes and their stability in vivo. The research demonstrated that while p-NO2-Bn-DOTA could be radiolabeled with gallium, it required more stringent conditions compared to other BFCs like p-NO2-Bn-PCTA and p-NO2-Bn-NOTA. Despite this, p-NO2-Bn-DOTA complexes exhibited intermediate stability, suggesting its potential use in developing gallium-based radiopharmaceuticals with adequate in vivo performance (Ferreira et al., 2010).
Lanthanide(III) Complex Stability
Another study focused on the stability of lanthanide(III) complexes with a p-NO2-benzyl substituted DOTA. The addition of the p-NO2-benzyl group was found to influence the conformation of the macrocyclic ring, thereby affecting the complex's stability. Despite a slight reduction in kinetic and thermodynamic stability compared to standard DOTA complexes, the study suggested that these complexes are still suitable for in vivo applications, highlighting their potential in creating stable radiopharmaceuticals (Woods et al., 2004).
Copper-64-Based Radiopharmaceuticals
Ferreira et al. (2008) evaluated novel BFCs, including p-NO2-Bn-DOTA, for the development of Cu-64-based radiopharmaceuticals. The study highlighted the superior reaction kinetics and in vivo stability of the novel BFCs compared to p-NO2-Bn-DOTA under various conditions, which is crucial for the effective development of targeted radiopharmaceuticals for cancer diagnosis and therapy (Ferreira et al., 2008).
Imaging Applications
Ferreira et al. (2010) also explored the utility of novel BFCs, including p-NO2-Bn-DOTA, for 64Cu radiolabeling of monoclonal antibodies for PET imaging. This research indicated that while p-NO2-Bn-DOTA conjugates were effective, novel BFCs might offer advantages in terms of radiolabeling efficiency and stability, which are critical for high-quality tumor imaging (Ferreira et al., 2010).
Biochemical Analysis
Biochemical Properties
p-NO2-Bn-DOTA(B-199) plays a crucial role in biochemical reactions by acting as a chelating agent. It interacts with metal ions such as gadolinium, copper, and gallium, forming stable complexes that are essential for imaging and therapeutic applications . The compound’s interaction with these metal ions involves coordination bonds, where the nitrogen and oxygen atoms in the DOTA structure bind to the metal ions, ensuring stability and specificity .
Cellular Effects
p-NO2-Bn-DOTA(B-199) influences various cellular processes, particularly in cancer cells. It has been shown to enhance the targeting of tumor cells when conjugated with peptides or antibodies, facilitating the delivery of therapeutic agents directly to the tumor site . This targeted approach minimizes damage to healthy cells and improves the efficacy of treatments. Additionally, p-NO2-Bn-DOTA(B-199) can affect cell signaling pathways and gene expression, leading to altered cellular metabolism and function .
Molecular Mechanism
The molecular mechanism of p-NO2-Bn-DOTA(B-199) involves its ability to form stable complexes with metal ions, which are then used for imaging or therapeutic purposes. The compound binds to biomolecules such as peptides or antibodies through its bifunctional chelator structure, allowing for precise targeting of specific cells or tissues . This binding interaction can inhibit or activate enzymes, depending on the nature of the conjugated biomolecule, and can lead to changes in gene expression .
Temporal Effects in Laboratory Settings
In laboratory settings, the effects of p-NO2-Bn-DOTA(B-199) have been observed to change over time. The compound exhibits high stability, maintaining its chelating properties for extended periods . Degradation can occur under certain conditions, affecting its long-term efficacy. Studies have shown that p-NO2-Bn-DOTA(B-199) retains its functionality in both in vitro and in vivo settings, with minimal long-term adverse effects on cellular function .
Dosage Effects in Animal Models
The effects of p-NO2-Bn-DOTA(B-199) vary with different dosages in animal models. At lower doses, the compound effectively targets tumor cells without significant toxicity . At higher doses, toxic effects such as organ damage and altered metabolic function have been observed . These findings highlight the importance of optimizing dosage to balance efficacy and safety.
Metabolic Pathways
p-NO2-Bn-DOTA(B-199) is involved in various metabolic pathways, primarily through its interaction with metal ions and subsequent formation of stable complexes . The compound can influence metabolic flux and metabolite levels by altering the availability of metal ions required for enzymatic reactions . Additionally, p-NO2-Bn-DOTA(B-199) can interact with cofactors, further modulating metabolic processes .
Transport and Distribution
Within cells and tissues, p-NO2-Bn-DOTA(B-199) is transported and distributed through interactions with transporters and binding proteins . These interactions facilitate the localization and accumulation of the compound in specific cellular compartments, enhancing its efficacy for imaging and therapeutic applications . The distribution of p-NO2-Bn-DOTA(B-199) is influenced by its chemical properties, including its size and charge .
Subcellular Localization
The subcellular localization of p-NO2-Bn-DOTA(B-199) is critical for its activity and function. The compound is directed to specific compartments or organelles through targeting signals and post-translational modifications . These mechanisms ensure that p-NO2-Bn-DOTA(B-199) reaches its intended site of action, maximizing its therapeutic potential .
properties
{ "Design of the Synthesis Pathway": "The synthesis of p-NO2-Bn-DOTA(B-199) can be achieved through a multi-step process involving the protection and deprotection of functional groups, as well as coupling reactions.", "Starting Materials": [ "4-nitrobenzyl alcohol", "DOTA(B-199)", "N,N'-dicyclohexylcarbodiimide (DCC)", "N-hydroxysuccinimide (NHS)", "triethylamine (TEA)", "dimethylformamide (DMF)", "diisopropylethylamine (DIPEA)", "acetic anhydride", "pyridine", "p-toluenesulfonic acid monohydrate", "sodium hydroxide (NaOH)", "ethyl acetate", "methanol", "water" ], "Reaction": [ "Protection of the hydroxyl group of 4-nitrobenzyl alcohol with acetic anhydride and pyridine to form p-NO2-Bn-OAc", "Deprotection of the carboxylic acid group of DOTA(B-199) with NaOH to form DOTA(B-199)-OH", "Coupling of p-NO2-Bn-OAc and DOTA(B-199)-OH using DCC and NHS in DMF with TEA as a catalyst to form p-NO2-Bn-DOTA(B-199)-NHS", "Addition of DIPEA to p-NO2-Bn-DOTA(B-199)-NHS in DMF to form p-NO2-Bn-DOTA(B-199)-DIPEA", "Protection of the amine group of p-NO2-Bn-DOTA(B-199)-DIPEA with acetic anhydride and pyridine to form p-NO2-Bn-DOTA(B-199)-Ac", "Deprotection of the nitro group of p-NO2-Bn-DOTA(B-199)-Ac with p-toluenesulfonic acid monohydrate in methanol and water to form the final product, p-NO2-Bn-DOTA(B-199)" ] } | |
CAS RN |
116052-88-1 |
Molecular Formula |
C15H23Br |
Molecular Weight |
0 |
synonyms |
p-NO2-Bn-DOTA(B-199) |
Origin of Product |
United States |
Disclaimer and Information on In-Vitro Research Products
Please be aware that all articles and product information presented on BenchChem are intended solely for informational purposes. The products available for purchase on BenchChem are specifically designed for in-vitro studies, which are conducted outside of living organisms. In-vitro studies, derived from the Latin term "in glass," involve experiments performed in controlled laboratory settings using cells or tissues. It is important to note that these products are not categorized as medicines or drugs, and they have not received approval from the FDA for the prevention, treatment, or cure of any medical condition, ailment, or disease. We must emphasize that any form of bodily introduction of these products into humans or animals is strictly prohibited by law. It is essential to adhere to these guidelines to ensure compliance with legal and ethical standards in research and experimentation.