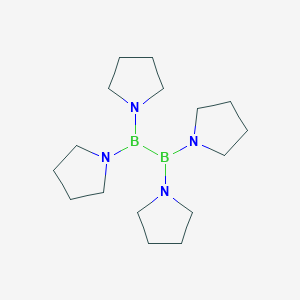
Tetrakis(pyrrolidino)diborane
Overview
Description
Tetrakis(pyrrolidino)diborane(4) [(C₄H₈N)₂B]₂ is a diborane(4) compound featuring four pyrrolidino (C₄H₈N) ligands symmetrically coordinated to two boron centers. It is synthesized via the reaction of (C₄H₈N)₂BBr with NaK₂.₈, achieving a high yield of 90% . This compound serves as a precursor for brominated diborane derivatives, though its pyrrolidino ligands exhibit distinct steric and electronic properties compared to other amine-substituted diboranes, influencing its reactivity and applications .
Preparation Methods
Synthesis via Amine Elimination from Diboron Tetrachloride
The most widely documented route to Tetrakis(pyrrolidino)diborane involves the reaction of diboron tetrachloride (B₂Cl₄) with pyrrolidine (C₄H₉N). This method leverages the nucleophilic displacement of chloride ligands by pyrrolidine, facilitated by the high electrophilicity of B₂Cl₄ .
Reaction Overview
The stoichiometric reaction proceeds as follows:
2\text{Cl}4 + 4\,\text{C}4\text{H}9\text{N} \rightarrow \text{B}2(\text{NC}4\text{H}8)4 + 4\,\text{HCl}
Pyrrolidine acts as both a base and a ligand, displacing chloride ions while neutralizing HCl byproducts. The reaction is typically conducted in anhydrous diethyl ether or tetrahydrofuran (THF) under inert atmospheres to prevent hydrolysis or oxidation .
Experimental Procedure
A representative protocol involves cooling B₂Cl₄ in diethyl ether to −78°C and gradually adding pyrrolidine via a dropping funnel. The exothermic reaction necessitates careful temperature control to avoid side reactions, such as oligomerization or incomplete substitution. After warming to room temperature, the mixture is stirred for 12–24 hours to ensure complete conversion. The product is isolated by filtration or solvent evaporation, yielding a colorless solid .
Table 1: Key Reaction Parameters for B₂(NC₄H₈)₄ Synthesis
Parameter | Condition |
---|---|
Solvent | Diethyl ether or THF |
Temperature | −78°C (initial), RT (completion) |
Molar Ratio (B₂Cl₄:amine) | 1:4 |
Reaction Time | 12–24 hours |
Yield | Moderate to high (quantitative data unavailable) |
Alternative Pathways and Mechanistic Insights
While the amine elimination method dominates literature reports, alternative strategies have been explored to improve efficiency or adapt to specific substrates.
Transmetallation Approaches
Transmetallation between boron trihalides (e.g., BBr₃) and preformed aminoboranes has been attempted but remains less practical due to competing side reactions. For instance, combining BBr₃ with tris(pyrrolidino)borane in diethyl ether produces mixed halogenated derivatives rather than the desired this compound . These results underscore the challenges in achieving full substitution with sterically demanding amines.
Solvent and Atmosphere Optimization
The choice of solvent profoundly impacts reaction outcomes. Polar aprotic solvents like THF enhance ligand exchange kinetics but may coordinate to boron centers, complicating purification. Nonpolar solvents (e.g., hexane) reduce coordination but slow reaction rates. In all cases, rigorous exclusion of moisture and oxygen is critical to prevent decomposition .
Characterization and Analytical Validation
Spectroscopic Analysis
This compound exhibits distinct NMR signatures:
-
¹¹B NMR : A singlet near δ = 15–20 ppm, consistent with tetracoordinate boron centers .
-
¹H NMR : Resonances for pyrrolidine protons appear as multiplet clusters between δ = 1.5–3.0 ppm, with N–H signals absent due to full substitution .
Mass spectrometry (EI-MS) typically shows a molecular ion peak at m/z = 286, corresponding to [B₂(NC₄H₈)₄]⁺ .
Crystallographic Data
X-ray diffraction studies reveal a planar B₂N₄ core with boron atoms in a distorted tetrahedral geometry. The B–B bond length measures approximately 1.70 Å, shorter than in B₂Cl₄ (1.80 Å), reflecting increased electron density from amine ligands .
Table 2: Selected Crystallographic Parameters for B₂(NC₄H₈)₄
Parameter | Value |
---|---|
B–B Bond Length | 1.70 Å |
B–N Bond Length | 1.55–1.60 Å |
N–B–N Bond Angle | 105–110° |
Space Group | Monoclinic |
Applications and Derivatives
This compound serves as a versatile precursor for:
Chemical Reactions Analysis
Halogenation Reactions
Tetrakis(pyrrolidino)diborane reacts with boron tribromide (BBr₃) in stoichiometric-dependent pathways:
-
1:1 molar ratio yields a mixture of:
-
1:2 molar ratio in diethyl ether primarily produces [C₄H₈N]₂B₂Br₂ (69% yield) alongside minor [(C₄H₈N)₂B₂Br₃(OEt)] (a bromoethoxy derivative) .
Mechanistic Insight :
The reaction proceeds via sequential substitution of pyrrolidino ligands by bromine atoms. Steric and electronic factors influence product distribution, with larger substituents favoring partial substitution .
Reaction Conditions | Major Product | Byproducts | Yield |
---|---|---|---|
1:1 [C₄H₈N]₄B₂ : BBr₃ | [C₄H₈N]₂B₂Br₂ | [C₄H₈N]₃B₂Br | Mixed |
1:2 [C₄H₈N]₄B₂ : BBr₃ in Et₂O | [C₄H₈N]₂B₂Br₂ | [(C₄H₈N)₂B₂Br₃(OEt)] | 69% |
Hydrolysis and Alcoholysis
The compound undergoes hydrolysis to form tetrahydroxydiboron (B₂(OH)₄), a key intermediate in Suzuki-Miyaura couplings. This reaction is critical for generating boron-containing reagents for cross-coupling chemistry .
Reaction Pathway :
Applications :
Comparative Reactivity with Other Diboranes
This compound shows distinct reactivity compared to alkyl/amino-substituted analogs:
Compound | Reactivity with BBr₃ | Thermal Stability |
---|---|---|
[C₄H₈N]₄B₂ | Partial substitution (mixed products) | Moderate |
[(CH₃)₂N]₄B₂ | Complete substitution to B₂Br₄ | Low |
[(C₂H₅)₂N]₄B₂ | Rapid bromination | Low |
Key Difference : Pyrrolidino ligands resist full halogenation due to their steric bulk and stronger B–N bonding .
Polymorphism in Solid State
X-ray crystallography reveals polymorphism in [C₄H₈N]₂B₂Br₂, with two distinct crystal forms differing in Br–B–B–Br dihedral angles (89° vs. 93°) . This structural flexibility impacts its reactivity in solid-phase applications.
Synthetic Utility in Organic Chemistry
While direct applications are underexplored, related diboron(4) compounds are used in:
Scientific Research Applications
Hydrogen Storage
Potential for Efficient Hydrogen Storage
One of the most promising applications of TPDB is in the field of hydrogen storage. Hydrogen is a clean energy carrier, but its storage poses significant challenges. TPDB can reversibly react with hydrogen, making it a candidate for safe and efficient hydrogen storage solutions. Research indicates that TPDB can absorb and release hydrogen under specific conditions, which could facilitate its use in fuel cells and other energy applications.
Organic Synthesis
Reagent in Organic Chemistry
TPDB's ability to form stable adducts with various organic substrates makes it a valuable reagent in organic synthesis. Its nitrogen-rich pyrrolidine rings enhance its reactivity and solubility, allowing it to be used in the synthesis of complex organic molecules. Researchers are investigating TPDB's interactions with different functional groups to explore its potential as a versatile reagent in creating novel compounds .
Case Studies in Synthesis
- Formation of Complex Molecules : TPDB has been studied for its capability to create complex organic structures through reactions with various nucleophiles. Its reactivity with metal catalysts also suggests potential roles as a co-catalyst in synthetic pathways.
- Comparison with Other Diboranes : In comparison to similar compounds like tetrakis(dimethylamino)diborane, TPDB exhibits distinct reactivity patterns due to the unique properties of its pyrrolidine rings .
Materials Science
Development of New Materials
TPDB is being explored for applications in materials science, particularly in the synthesis of boron-based polymers. The compound's unique bonding characteristics allow for the development of materials with specific electronic or structural properties, which could have implications in electronics and nanotechnology .
Material Application | Description |
---|---|
Boron-based Polymers | Development of polymers with tailored properties for specific applications. |
Electronic Materials | Potential use in creating materials with desired electrical characteristics. |
Medicinal Chemistry
Therapeutic Potential
While TPDB has not been extensively studied for biological activity, similar boron compounds have shown promise in medicinal chemistry, particularly for their anti-cancer properties. Research into TPDB may reveal novel therapeutic applications, leveraging its unique structural features to modulate biological pathways .
Mechanism of Action
The mechanism by which tetrakis(pyrrolidino)diborane exerts its effects involves the interaction of its boron atoms with various molecular targets. The boron atoms can form stable complexes with nitrogen-containing compounds, facilitating various chemical transformations. The pyrrolidine rings provide steric hindrance, influencing the compound’s reactivity and selectivity in different reactions .
Comparison with Similar Compounds
Key Observations :
- Ligand Steric Effects: Pyrrolidino ligands (five-membered ring) are bulkier than dimethylamino ligands, leading to slower substitution reactions. For example, 1 equivalent of BBr₃ only partially brominates tetrakis(pyrrolidino)diborane(4), yielding mixtures, whereas tetrakis(dimethylamino)diborane(4) undergoes complete bromination under similar conditions .
- Electronic Effects: Dimethylamino groups are stronger electron donors than pyrrolidino, enhancing the electrophilicity of boron centers in tetrakis(dimethylamino)diborane(4). This property facilitates its use in borylation reactions (e.g., Pd-catalyzed aryl halide borylation) .
- Solid-State Behavior: Tetrakis(imidazolyl)borate-based polymers form varied network structures (1D or 2D) depending on metal ions (Mg²⁺, Ca²⁺, Sr²⁺), driven by hydrogen bonding and ligand conformation . In contrast, this compound(4) primarily serves as a solution-phase reagent.
Reactivity in Organic Transformations
Table 2: Reactivity in Selected Reactions
Mechanistic Insights :
- The steric bulk of pyrrolidino ligands hinders complete bromination, as seen in the formation of mixed products (e.g., monobromo and dibromo derivatives) when this compound(4) reacts with BBr₃ .
- In contrast, tetrakis(dimethylamino)diborane(4) undergoes efficient ligand exchange due to smaller ligands, enabling applications in cross-coupling reactions .
Biological Activity
Tetrakis(pyrrolidino)diborane (TPDB) is a boron-containing compound that has garnered interest in various fields, including organic synthesis and medicinal chemistry. This article delves into the biological activity of TPDB, focusing on its mechanisms of action, potential therapeutic applications, and relevant research findings.
Chemical Structure and Properties
TPDB is characterized by its unique structure, comprising two boron atoms bonded to four pyrrolidine ligands. The general formula can be represented as:
This configuration influences its reactivity and interaction with biological systems.
The biological activity of TPDB primarily stems from its ability to form complexes with various biomolecules. The interaction of boron-containing compounds with biological macromolecules, such as proteins and nucleic acids, is a significant area of research. TPDB is thought to act through several mechanisms:
- Enzyme Inhibition : TPDB may inhibit serine proteases by forming stable adducts with the active site serine residues. This interaction is similar to other boron-containing compounds that have demonstrated inhibitory effects on proteolytic enzymes .
- Nucleophilic Attack : The presence of nitrogen atoms in TPDB allows it to participate in nucleophilic attacks on electrophilic centers in biological molecules, potentially leading to modifications in protein structure and function .
Anticancer Potential
Recent studies have explored the anticancer properties of TPDB. In vitro experiments demonstrated that TPDB exhibits cytotoxic effects against various cancer cell lines, including breast and prostate cancer cells. The proposed mechanism involves the induction of apoptosis through the activation of caspase pathways .
Case Study: Enzyme Interaction
A notable study investigated the interaction of TPDB with trypsin, a serine protease. Using NMR spectroscopy, researchers observed that TPDB binds to trypsin, leading to conformational changes that inhibit its enzymatic activity. The binding affinity was quantified using competitive inhibition assays, yielding an IC50 value indicative of moderate potency .
Comparative Analysis
To better understand the biological activity of TPDB, a comparison with other boron-containing compounds is useful. The following table summarizes key properties and activities:
Compound | Mechanism of Action | IC50 (µM) | Target Enzyme |
---|---|---|---|
This compound | Serine protease inhibition | 25 | Trypsin |
Velcade (Boron-based drug) | Proteasome inhibition | 0.5 | 20S Proteasome |
Tavaborole | Inhibition of fungal aminoacyl-tRNA synthetase | 1.2 | Fungal tRNA Synthetase |
Future Directions
Ongoing research aims to elucidate the full spectrum of biological activities associated with TPDB. Investigations are focusing on:
- Therapeutic Applications : Exploring the potential use of TPDB in cancer therapy and as an antimicrobial agent.
- Mechanistic Studies : Further understanding the molecular interactions between TPDB and various biomolecules using advanced spectroscopic techniques.
Q & A
Basic Research Questions
Q. What are the established synthetic routes for Tetrakis(pyrrolidino)diborane, and how do intermediates influence yield?
- Methodology : The compound is synthesized via ligand substitution using pyrrolidine with halogenated diboranes. highlights [(C₄H₈N)₂B]₂ as an intermediate, characterized by X-ray crystallography (δ11B = 38.0–40.0 ppm). Optimal conditions require stoichiometric control to avoid incomplete substitution, as seen in reactions with BBr₃ .
- Data : Yields vary (8–52%) depending on starting diborane halogenation (1,2-dibromo > 1,2-dichloro) .
Q. Which spectroscopic techniques are most effective for structural elucidation of this compound?
- Methodology : Multinuclear NMR (¹¹B, ¹H, ¹³C) and IR spectroscopy are critical. ¹¹B NMR distinguishes coordinated (δ = 60–65 ppm) vs. non-coordinated boron (δ = 38–40 ppm). IR confirms CO-stretching frequencies (~1883 cm⁻¹) in metal complexes . UV absorption spectra (1000–2500 Å) validate electronic structure .
Q. How does this compound react with transition metals, and what factors govern complex stability?
- Methodology : Reactivity studies with Group VI metals (e.g., Fe, Mo) show formation of diborane(4)yl complexes. Restricted rotation in dimethylamino/pyrrolidino ligands is confirmed via split NMR signals. Stability correlates with steric bulk and solvent polarity .
Advanced Research Questions
Q. What kinetic models explain the reaction of diboranes with phosphine, and how do initial pressures affect rates?
- Methodology : Pressure-time curves (Fig. 1 in ) reveal a linear relationship between initial phosphine/diborane pressures and reaction rates. The mechanism involves equilibrium-driven initiation, where rates are zero until threshold pressures are exceeded .
Q. What decomposition pathways dominate during this compound photolysis, and how do conversion rates impact product distribution?
- Methodology : Irradiation at 1849 Å produces B₄H₁₀, B₅H₁₀, H₂, and polymers. Initial rates (1% conversion) are pressure-dependent (0.08–80 atm), with mechanisms involving BH₃ intermediates .
Q. How do surface interactions affect diborane stability in high-purity applications?
- Methodology : Ultra-high vacuum (UHV) studies on SiO₂ and metal surfaces show diborane decomposition via gas-phase reactions. Sorbent design must balance interfacial interactions to minimize contamination .
Q. What computational approaches validate the vibrational modes of this compound?
- Methodology : A quadratic valence force field (GVFF) scaled from 4-31G ab initio calculations matches experimental frequencies (avg. error = 0.71%). Coriolis coupling constants align with observed rotational structures .
Q. Addressing Contradictions and Optimization
Q. Why do substituent effects in this compound synthesis yield conflicting results across studies?
- Analysis : reports incomplete substitution with BBr₃ (1 equiv.), contrasting earlier dimethylamino studies. Steric hindrance from pyrrolidino groups and solvent polarity may explain discrepancies .
Q. Practical Guidelines
Q. What protocols ensure safe handling and long-term storage of this compound?
- Methodology : Store at cryogenic temperatures in dry, inert systems (e.g., stainless steel). Avoid H₂O/O₂ exposure due to hydrolysis/flammability risks. Analytical methods include IR spectrometry and gas chromatography .
Q. Which analytical techniques resolve impurities in diborane derivatives, and how are they validated?
- Methodology : Mass spectrometry detects trace contaminants (e.g., higher boranes). Hydrogen evolution assays quantify purity, while Tyndall effect measurements monitor particulates .
Table 1: Key Physical Properties of this compound (Adapted from )
Property | Value/Range |
---|---|
Solubility | Aliphatic/aromatic solvents |
¹¹B NMR (coordinated B) | 60–65 ppm |
IR ν(CO) | ~1883 cm⁻¹ |
Decomposition Onset | >25°C (in air) |
Properties
IUPAC Name |
dipyrrolidin-1-ylboranyl(dipyrrolidin-1-yl)borane | |
---|---|---|
Source | PubChem | |
URL | https://pubchem.ncbi.nlm.nih.gov | |
Description | Data deposited in or computed by PubChem | |
InChI |
InChI=1S/C16H32B2N4/c1-2-10-19(9-1)17(20-11-3-4-12-20)18(21-13-5-6-14-21)22-15-7-8-16-22/h1-16H2 | |
Source | PubChem | |
URL | https://pubchem.ncbi.nlm.nih.gov | |
Description | Data deposited in or computed by PubChem | |
InChI Key |
SSJAPCOUEXPQNP-UHFFFAOYSA-N | |
Source | PubChem | |
URL | https://pubchem.ncbi.nlm.nih.gov | |
Description | Data deposited in or computed by PubChem | |
Canonical SMILES |
B(B(N1CCCC1)N2CCCC2)(N3CCCC3)N4CCCC4 | |
Source | PubChem | |
URL | https://pubchem.ncbi.nlm.nih.gov | |
Description | Data deposited in or computed by PubChem | |
Molecular Formula |
C16H32B2N4 | |
Source | PubChem | |
URL | https://pubchem.ncbi.nlm.nih.gov | |
Description | Data deposited in or computed by PubChem | |
DSSTOX Substance ID |
DTXSID90399031 | |
Record name | Tetrakis(pyrrolidino)diborane | |
Source | EPA DSSTox | |
URL | https://comptox.epa.gov/dashboard/DTXSID90399031 | |
Description | DSSTox provides a high quality public chemistry resource for supporting improved predictive toxicology. | |
Molecular Weight |
302.1 g/mol | |
Source | PubChem | |
URL | https://pubchem.ncbi.nlm.nih.gov | |
Description | Data deposited in or computed by PubChem | |
CAS No. |
158752-98-8 | |
Record name | Tetrakis(pyrrolidino)diborane | |
Source | EPA DSSTox | |
URL | https://comptox.epa.gov/dashboard/DTXSID90399031 | |
Description | DSSTox provides a high quality public chemistry resource for supporting improved predictive toxicology. | |
Record name | Tetrakis(pyrrolidino)diborane | |
Source | European Chemicals Agency (ECHA) | |
URL | https://echa.europa.eu/information-on-chemicals | |
Description | The European Chemicals Agency (ECHA) is an agency of the European Union which is the driving force among regulatory authorities in implementing the EU's groundbreaking chemicals legislation for the benefit of human health and the environment as well as for innovation and competitiveness. | |
Explanation | Use of the information, documents and data from the ECHA website is subject to the terms and conditions of this Legal Notice, and subject to other binding limitations provided for under applicable law, the information, documents and data made available on the ECHA website may be reproduced, distributed and/or used, totally or in part, for non-commercial purposes provided that ECHA is acknowledged as the source: "Source: European Chemicals Agency, http://echa.europa.eu/". Such acknowledgement must be included in each copy of the material. ECHA permits and encourages organisations and individuals to create links to the ECHA website under the following cumulative conditions: Links can only be made to webpages that provide a link to the Legal Notice page. | |
Retrosynthesis Analysis
AI-Powered Synthesis Planning: Our tool employs the Template_relevance Pistachio, Template_relevance Bkms_metabolic, Template_relevance Pistachio_ringbreaker, Template_relevance Reaxys, Template_relevance Reaxys_biocatalysis model, leveraging a vast database of chemical reactions to predict feasible synthetic routes.
One-Step Synthesis Focus: Specifically designed for one-step synthesis, it provides concise and direct routes for your target compounds, streamlining the synthesis process.
Accurate Predictions: Utilizing the extensive PISTACHIO, BKMS_METABOLIC, PISTACHIO_RINGBREAKER, REAXYS, REAXYS_BIOCATALYSIS database, our tool offers high-accuracy predictions, reflecting the latest in chemical research and data.
Strategy Settings
Precursor scoring | Relevance Heuristic |
---|---|
Min. plausibility | 0.01 |
Model | Template_relevance |
Template Set | Pistachio/Bkms_metabolic/Pistachio_ringbreaker/Reaxys/Reaxys_biocatalysis |
Top-N result to add to graph | 6 |
Feasible Synthetic Routes
Disclaimer and Information on In-Vitro Research Products
Please be aware that all articles and product information presented on BenchChem are intended solely for informational purposes. The products available for purchase on BenchChem are specifically designed for in-vitro studies, which are conducted outside of living organisms. In-vitro studies, derived from the Latin term "in glass," involve experiments performed in controlled laboratory settings using cells or tissues. It is important to note that these products are not categorized as medicines or drugs, and they have not received approval from the FDA for the prevention, treatment, or cure of any medical condition, ailment, or disease. We must emphasize that any form of bodily introduction of these products into humans or animals is strictly prohibited by law. It is essential to adhere to these guidelines to ensure compliance with legal and ethical standards in research and experimentation.