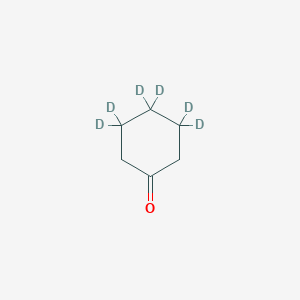
Cyclohexanone-3,3,4,4,5,5-d6
Overview
Description
Cyclohexanone-3,3,4,4,5,5-d6, also known as Cyclohexanone-d6, is a stable isotope labelled compound . It has a molecular formula of C6 D6 H4 O and a molecular weight of 104.180 .
Molecular Structure Analysis
The molecular structure of Cyclohexanone-3,3,4,4,5,5-d6 is represented by the SMILES notation [2H]C1 ( [2H])CC (=O)CC ( [2H]) ( [2H])C1 ( [2H]) [2H] and the InChI notation InChI=1S/C6H10O/c7-6-4-2-1-3-5-6/h1-5H2/i1D2,2D2,3D2 .Physical And Chemical Properties Analysis
Cyclohexanone-3,3,4,4,5,5-d6 has a molecular formula of C6 D6 H4 O and a molecular weight of 104.180 .Scientific Research Applications
Conformational Features and Stability
Cyclohexanone-3,3,4,4,5,5-d6, like its parent compound cyclohexanone, has unique conformational features. It exists almost exclusively in the chair form, with only a small amount in skew–boat forms . The conformational features of cyclohexanone are shown briefly in the Figure . The chair conformation has only a vertical σ-plane passing through C1 and C4 and belongs to the point group CS .
Stereochemical Aspects
The stereochemical aspects of the addition of nucleophiles to cyclohexanones have been studied extensively . This includes PDC (PSC) and SAC (SSC) effects . The torsional strain and the role of C-2 and C-6 axial hydrogens are also of interest .
HIV-1 Integrase Inhibitors
Derivatives of cyclohexanone, such as 2,6-bis(3,4,5-trihydroxybenzylydene) derivatives, have been synthesized and tested as HIV-1 integrase (IN) inhibitors . These compounds have shown the ability to inhibit both 30 -processing and disintegration .
Anti-topoisomerase Activity
Some derivatives of cyclohexanone have been reported to have antitopoisomerase activity . This is particularly relevant in the context of cancer research, as topoisomerases are enzymes that are involved in the overwinding or underwinding of DNA .
Synthesis of Solenopsin A
The synthesis of Solenopsin A involves the application of A (1,2) Strain Concept . This is a significant application in the field of organic synthesis .
Catalysts and Solvents
Cyclohexanone and its derivatives have been used as catalysts and solvents in various chemical reactions . This includes reactions involving ionic liquids .
Safety and Hazards
properties
IUPAC Name |
3,3,4,4,5,5-hexadeuteriocyclohexan-1-one | |
---|---|---|
Source | PubChem | |
URL | https://pubchem.ncbi.nlm.nih.gov | |
Description | Data deposited in or computed by PubChem | |
InChI |
InChI=1S/C6H10O/c7-6-4-2-1-3-5-6/h1-5H2/i1D2,2D2,3D2 | |
Source | PubChem | |
URL | https://pubchem.ncbi.nlm.nih.gov | |
Description | Data deposited in or computed by PubChem | |
InChI Key |
JHIVVAPYMSGYDF-NMFSSPJFSA-N | |
Source | PubChem | |
URL | https://pubchem.ncbi.nlm.nih.gov | |
Description | Data deposited in or computed by PubChem | |
Canonical SMILES |
C1CCC(=O)CC1 | |
Source | PubChem | |
URL | https://pubchem.ncbi.nlm.nih.gov | |
Description | Data deposited in or computed by PubChem | |
Isomeric SMILES |
[2H]C1(CC(=O)CC(C1([2H])[2H])([2H])[2H])[2H] | |
Source | PubChem | |
URL | https://pubchem.ncbi.nlm.nih.gov | |
Description | Data deposited in or computed by PubChem | |
Molecular Formula |
C6H10O | |
Source | PubChem | |
URL | https://pubchem.ncbi.nlm.nih.gov | |
Description | Data deposited in or computed by PubChem | |
DSSTOX Substance ID |
DTXSID90514641 | |
Record name | (3,3,4,4,5,5-~2~H_6_)Cyclohexanone | |
Source | EPA DSSTox | |
URL | https://comptox.epa.gov/dashboard/DTXSID90514641 | |
Description | DSSTox provides a high quality public chemistry resource for supporting improved predictive toxicology. | |
Molecular Weight |
104.18 g/mol | |
Source | PubChem | |
URL | https://pubchem.ncbi.nlm.nih.gov | |
Description | Data deposited in or computed by PubChem | |
Product Name |
Cyclohexanone-3,3,4,4,5,5-d6 | |
CAS RN |
54513-99-4 | |
Record name | (3,3,4,4,5,5-~2~H_6_)Cyclohexanone | |
Source | EPA DSSTox | |
URL | https://comptox.epa.gov/dashboard/DTXSID90514641 | |
Description | DSSTox provides a high quality public chemistry resource for supporting improved predictive toxicology. | |
Synthesis routes and methods I
Procedure details
Synthesis routes and methods II
Procedure details
Disclaimer and Information on In-Vitro Research Products
Please be aware that all articles and product information presented on BenchChem are intended solely for informational purposes. The products available for purchase on BenchChem are specifically designed for in-vitro studies, which are conducted outside of living organisms. In-vitro studies, derived from the Latin term "in glass," involve experiments performed in controlled laboratory settings using cells or tissues. It is important to note that these products are not categorized as medicines or drugs, and they have not received approval from the FDA for the prevention, treatment, or cure of any medical condition, ailment, or disease. We must emphasize that any form of bodily introduction of these products into humans or animals is strictly prohibited by law. It is essential to adhere to these guidelines to ensure compliance with legal and ethical standards in research and experimentation.